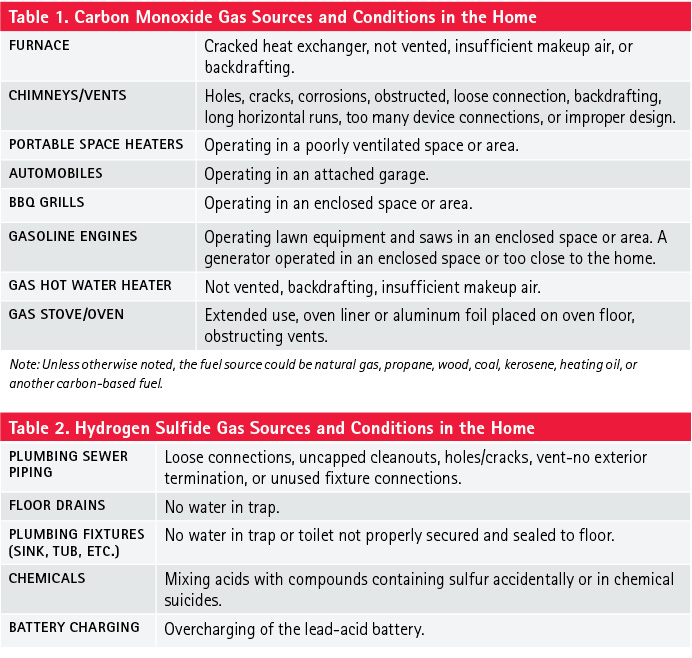
A survey of 185 first responders from career and volunteer fire departments in the southeastern region of the United Stated relative to four-gas monitors was conducted by the University of North Carolina—Charlotte in 2014. Respondents indicated a lack of understanding pertaining to instrument response readings of parts per million (ppm) and percent volume (% vol), instrument alarms, and type of calibration gases. Survey participants recommended that each department assess its capabilities and needs relative to training personnel on the use and interpretation of instrument response readings.
RELATED FIREFIGHTER TRAINING
Wireless Gas Detection Systems
FIXED-POINT GAS DETECTION SYSTEMS
This article explains how to interpret readings from instruments that contain oxygen, percent of lower explosive level (% LEL) flammable gas/vapor, carbon monoxide (CO), and hydrogen sulfide (H2S) sensors. These sensors are typically found in the multiple gas detectors commonly used by the fire service.
Components for Interpreting Readings
Four components will assist in interpreting instrument response readings. The reading is only one part of the gas detection puzzle. The other components are people, sources, and conditions. Taken together with the instrument response readings, these components can help you assess an incident scene and develop and implement an action plan.
People. People provide clues or information through exposure health effects, actions, behaviors, identifying locations, and material information. They could be the persons exposed, witnesses, company representatives, or others who may respond to aid such as a hazmat response team (HMRT) or gas utility company representative.
People play a key role in initiating the need for fire department services and completing the puzzle in the initial and ongoing stages of an incident. In many instances, they are the initial starting point of the puzzle. The primary people in this component are those showing exposure signs and symptoms. People exposed to a material should be evaluated by emergency medical services (EMS). EMS can assist with performing a more systematic and thorough evaluation of exposure signs and symptoms. Information obtained by EMS personnel can be used in assessing and classifying or identifying the material. Eyewitnesses, company representatives, and others who respond, such as an HMRT or a gas utility company representative, also contribute to this component. They may be able to identify the source and condition.
However, there will be times when you must piece information together by interviewing people to obtain preincident and postincident information on exposure signs/symptoms, occurrences, actions, behaviors, and observations. This will help with developing a timeline and completing the puzzle.
Gas utility provider personnel respond along with the fire department on natural gas leaks. These representatives have additional expertise on leak detection and hazard assessment. Their detection equipment will be specific to the gas they wish to detect (e.g., methane). Their instruments are often more sensitive and can detect lower levels of a gas or vapor than the detection instruments used by the fire department—sometimes, concentrations that will not register on fire department instruments. This usually leads to a misconception that the instrument being used is not operating properly, which is not true if the instrument is properly maintained and calibrated.
Depending on the local protocol, the availability of the team, and the type or severity of the incident, an HMRT may respond as part of the initial call or if requested. HMRTs are equipped with other detection and sampling instruments not carried by a standard firefighting crew. These instruments can confirm the initial assessment and identify or classify a material that your instrument is not detecting. HMRTs also have instruments that can sample materials at their source of normal physical state (solid, liquid, or gas) to further evaluate a material. Hazmat technicians have additional training on chemical and physical properties, containers, and identification of sources and conditions.
Sources. A source is a thing or place from which a gas or vapor is released or emitted. The source can be an occupancy, a container (a tank or cylinder, for example), piping, sewers, sumps, waste receptacles, heating equipment, stoves, ovens, chimneys, vents, confined spaces, batteries, vehicles, or machinery. We can apply the basic clues of occupancy/location, container types/shapes, markings/colors, placards/labels, shipping papers, detection instruments, visual indicators of a spill or leak, and our senses to identify hazardous materials and their sources. Once sources have been identified, they need to be assessed for conditions to determine if the source is the cause of the airborne contaminant being released.
Conditions. Conditions refer to the occurrence or failure that causes a vapor or gas to be released into an area, exposing people, property, or the environment to a hazard. The hazard could be fire/explosion, corrosive, toxic, or inert; it can also be accidental or nonaccidental. If the condition is not identified through the interview process, you will have to assess the sources for the conditions. You can assess and identify conditions by using your senses, instrument response, and thermal imaging cameras (TICs). Tables 1 and 2 are examples of sources and conditions of CO and H2S gases, respectively, in the home.
Visual observation is the most common assessment method. Visually assess the area around the source signs of spills or leaks. Look for deterioration, discoloration, holes, openings, loose connections, or open valves that would allow the material to escape. A TIC can enhance or supplement your visual assessment and indicate temperature levels. Chemicals undergoing a reaction will generate a change in temperature that can be identified and monitored, and you can determine the level of material in an uninsulated container. The instrument cannot be used on a container that has a reflective surface. Liquid leak detection solutions can be used to help identify leaks from piping, valves, and containers. Use a commercial grade leak detection solution as opposed to a soap and water mixture that can corrode threads and piping. Hissing sounds or other similar noises could be indications of a leak under pressure from a container or pipe. Limit using your nose for odor detection because of potential health risks; keep in mind also that many chemicals are odorless. A person exposed to a material could provide insight relative to the odor, which can be matched with a specific material. An odor does not always ensure detection because many material odor thresholds are below the detector’s minimum detection limits.
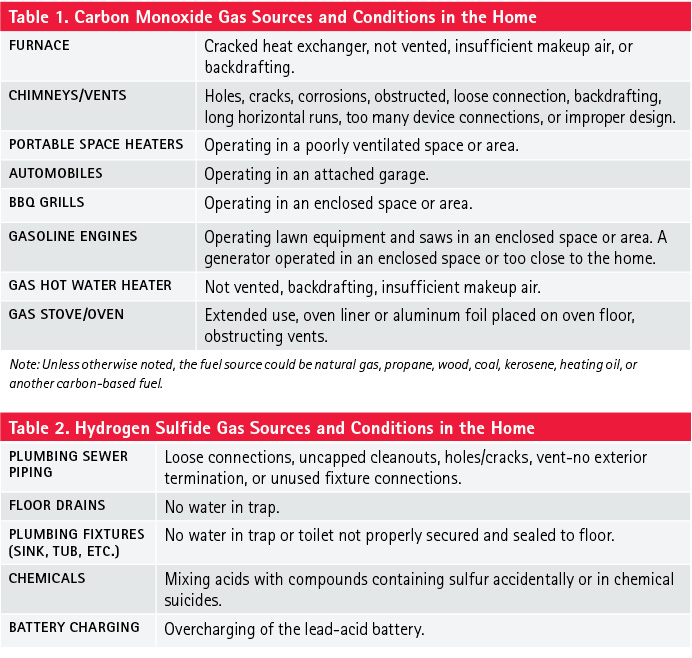
Instrument Response Readings
Instrument response is a readout of one or more sensors that indicates an airborne gas or vapor is in the surrounding atmosphere. To obtain a reading, assess the areas of the incident in a systematic manner to expose the instrument to the gases or vapors. If available, use two instruments; this will help you cover more area and provide a redundancy of readings to compare. You must determine the areas or locations from which to take the air samples for assessment.
Sampling points—the general locations or areas of the incident—are dynamic and influenced by factors such as physical and chemical properties and weather conditions. Assess the areas of command, apparatus, and personnel staging to ensure the safety of all responders. Establish a 360° isolation perimeter, starting downwind. If the incident occurs inside a building, obtain an instrument reading at the doorway before entering the building. You may have to break locations down into rooms, floors, or quadrants to narrow down the source and condition. Obtain instrument readings adjacent to (within five feet of) identified sources and conditions. The monitor sensor inlet point must be directly in the gas or vapor space, concentration, or accumulation to produce an instrument reading representative of the gas or vapor concentration in the instrument’s immediate vicinity.
Sample and evaluate multiple sampling points. Interior rooms and floors can have different concentrations of gases or vapors. Exterior areas, particularly where the gases or vapors collect such as low spots and physical barriers, can have areas with higher concentrations of gases or vapors. Frequency and multiple sampling points help to identify trends—areas with higher or lower readings or that increase or decrease based on an area or incident time frame.
If the material is suspected or unidentified, position the instrument to sample low, middle, and high horizontal zones at each sampling point—all three horizontal zones need to be assessed. If the material is positively identified, assess the horizontal zone based on the material’s vapor density. Identifying the horizontal zone where the gas or vapor is collecting will help you classify or identify a material. Propane, butane, and flammable liquid vapors have vapor densities heavier than air, necessitating that the middle to low horizontal zones be evaluated. Natural gas (methane) has a vapor density lighter than air, which necessitates that the middle to high zones be evaluated. When checking high and low zones, obtain a reading as close as possible to the surface (floor or ceiling surfaces) to ensure you are getting a reading of the gas or vapor that may be collecting near the surface and spreading out.
Just walking through an area will not indicate whether gases or vapors are present in the surrounding atmosphere. Stop at each sampling point to obtain an accurate reading. When using the instrument at a specific sampling point, consider instrument lag times, which consist of response time and recovery time. Response time is the time in seconds from when the material enters the sensor and a reading is displayed on the instrument screen. Response time depends on the sensor type. Consult the instrument manufacturer’s specifications to determine the response times for each sensor in the instrument. The response time is indicated by “t90” with the accompanying time in seconds in the instrument manual. This refers to the time that it takes the instrument to obtain a 90% reading of the airborne gases or vapors. Generally, response time is 15 to 30 seconds. When using a sampling probe and hose, add an additional two seconds for every foot of total assembly to the response time. If there is an instrument response, time is needed to allow the instrument to clear the previous reading and to produce a new reading at a different sampling point. This is known as recovery time. Recovery time could be 60 seconds or more, depending on the sensor type, material type and concentration, and if a sampling probe and hose are used.
Once exposed to gases or vapors, an interaction with the sensors will produce a signal that is processed with the instrument electronics to display an instrument response. This is the extent of the “intelligence” within the instrument. The instrument cannot positively identify the material and make decisions as to what actions need to be taken based on the instrument response. Until the material is positively identified with correlating information from the other components of the puzzle, the instrument response reading is an indication of the presence of a material that is interacting with a sensor. It is up to the user to interpret the readings and to verify and make decisions as to actions to take. You must use your experience and knowledge to interpret the instrument response and apply other components of the puzzle to form a conclusion.
Evaluate as part of your interpretation the type and number of sensor instrument readings and the reading levels at each sampling point. Substance-specific sensors such as CO and H2S can detect and respond to other gases or vapors in an environment.
A gas or vapor that is not the target material that produces an instrument response is known as cross-sensitivity. All sensors are subject to cross-sensitivity, which can influence instrument response readings. Cross-sensitivity can produce a positive or a negative number reading. A cross-sensitivity vapor or gas in the presence of a target can increase or decrease readings depending on the material. Most manufacturers publish cross-sensitivity information for their instrument sensors. It is important to know and understand these cross-sensitivities and keep the information with the instrument on the apparatus. Consider cross-sensitivity if the source or condition information is not correlating with the instrument response readings, if there is more than one sensor reading, or if there is a negative sensor reading. A negative instrument response reading could also be an indication that the zeroing or fresh air setup was not done in a clean environment.
No Response Readings
If no response readings are produced, consider the following factors:
- The gas or vapor may not be in the area of the sampling point. Ensure that the instrument sensor inlet point is directly in the gas or vapor space, concentration, or accumulation. The instrument response readings are representative of the gas or vapor concentration in the immediate vicinity of the monitor. Sample and evaluate multiple sampling points.
- The instrument may not have the correct sensor for the gas or vapor present. Other sensor types or technologies may be needed to thoroughly evaluate the environment. Use another instrument; you can obtain one from an HMRT or other technical specialist.
- The concentration of contaminants may be below the sensor’s minimum detection level. The odor threshold of a material can be well below the minimum detection limit of a sensor. Since calling 911, the gases or vapors may have dissipated because of ventilation performed by the caller or the material dissipated from its container or source and is no longer present. The building may have to be closed to allow for the gases or vapors to build up to be detected by the instrument.
Even if no instrument responses are produced, the incident must be thoroughly investigated. “No instrument responses” should not be the main decision for terminating an incident. All pieces of the puzzle need to be thoroughly investigated before terminating the incident. After a thorough investigation, “no response” should be noted in the documentation as opposed to using zero for noninstrument responses.
Detection and Scene Size-Up
Initial assessment of an incident and the application of portable gas detection can be categorized as “known,” “suspected,” or “unidentified”:
A known incident type denotes that the material is readily and positively identified in the initial scene size-up. An incident involving a natural gas line struck by an excavator is an example.
A suspected incident type denotes there is reasonable suspicion of the identification of the material involved. A household CO detector alarm activation is an example. A suspected incident requires putting together pieces of the puzzle to verify and support your initial assessment. Be careful. Do not become fixated on your initial assessment in a suspected incident and become blind to other potential sources or conditions.
An unidentified incident type has limited clues or information on the identification of the source and condition. An odor investigation call is an example of an unidentified incident type. An unidentified incident scenario is more challenging and necessitates a more thorough assessment of the scene. An HMRT or another technical specialist or outside agency may be needed to assist. Additional detection and sampling equipment may also be needed. No matter what the incident type, you must be diligent and systematic in your assessment.
Fitting the Pieces Together
Interpreting instrument response readings and making decisions necessitate putting together all pieces of the puzzle. The process is continuous and dynamic; existing information is confirmed, and new information is added as the incident progresses. Clues and information obtained under each of the four components need to confirm and support each other. Avoid presumptions of the incident that could cause you to overlook or misinterpret clues and information. If clues and information do not line up, you need to ask why, continue your investigation, and do the following:
- Revaluate identified sources and conditions.
- Reassess for unidentified sources and conditions.
- Identify and evaluate new sampling points.
- Use another instrument or different sensor technology.
- Consider sensor cross-sensitivity.
- Recalibrate the instrument.
- Consult with an HMRT or another technical specialist.
Identifying incident facts and trends within the four components discussed above are an important part of the hazard assessment process. Potential trends may include the following: Are there changes or differences of instrument responses readings from one sampling point to another? Are there consistent instrument response readings or other areas with higher readings than others? Is the gas or vapor spreading or receding in relation to the identified source and condition? Are new people reporting exposure signs and symptoms? Is the number of people exposed to the material increasing? Are the exposure signs and symptoms of those exposed improving or getting worse?
Putting Meaning to Numbers
The use and interpretation of an instrument response reading are only as effective as your ability to identify and assess exposure signs/symptoms, sources, and conditions. Until the material is positively identified with correlating information from the other components of the puzzle, the instrument response reading is only an indication of the presence of a material that is interacting with the sensors. Interpreting readings is essential for assessing public exposure, personal protective equipment requirements, and incident mitigation. If firefighters do not understand why or how an instrument response is being produced, they may not trust the instrument and may disregard the reading, which could lead to poor tactical decisions or worse.
Defining an instrument reading depends on the sensor type (i.e., oxygen, flammable, or toxic) and correlating the number with published health effects, exposure limits, or action levels. Exposure limits are the maximum amount or concentration of a chemical that a person can be exposed to during a period. Exposure limits are based on either a regulatory requirement or best practices. Action levels are response readings to a known or an unknown airborne contaminant that trigger some action. The action could be ventilation, don self-contained breathing apparatus, evacuate occupants, or remove firefighters. A regulator agency standard or local protocol may trigger an action level.
Oxygen sensor readings are measured in percent volume (% vol)—a percentage of measurement of a gas or vapor in a volume of a concentration. CO, H2S, and other substance-specific toxic electrochemical sensor readings are measured in ppm; 1% vol is equal to 10,000 ppm. For example, if a jar contains a million jellybeans and 20,000 of these jellybeans are purple, this is equal to 20,000 ppm or 2% vol of purple jellybeans in the jar. Flammable gas and vapor readings are commonly measured in percent lower explosive limit (% LEL)—a measurement of the range between 0% vol and the % LEL point.
Instruments have alarms that are audible, visual, and tactile to alert the user that a specific reading level has been achieved. Alarm set points are based on the specific hazard—oxygen, fire/explosion, and toxic. Substance-specific toxic sensor alarms are specific to a material. Most instruments have low, high, short-term exposure limit (STEL), and time-weighted average (TWA) alarms. Most instrument manufacturers do not differentiate between the alarm types other that what is shown on the instrument screen readout.
You need to know the specific action required if an alarm sounds. These actions could be to evacuate the area, don respiratory protection, or implement mitigation practices such as ventilation. These actions should be identified in standard operating procedures and training programs. Alarm set points are based on exposure limits [e.g., Threshold Limit Value/TWA, immediately dangerous to life or health (IDLH)] established government regulatory standards and nonregulatory organizations.
Toxic Sensor Response Readings
The operational range of CO, H2S, and other substance-specific toxic sensors varies by the sensor type and manufacturer. Most toxic sensor readings are in 1-ppm increments and have a minimum detection limit of 1 ppm.
The health effects of a gas or vapor depend on the concentration and length of exposure. The exposed person’s age and health history also influence the effects and need to be considered. Acute and chronic exposures need to be evaluated when interpreting toxic response readings. Developing a timeline of an exposure time range will help with the assessment of possible chronic exposures.
Oxygen Sensor Response Readings
The atmosphere is made up of 20.9% oxygen; 78% nitrogen; 1.1% argon; and trace amounts of carbon dioxide, neon, and helium. 1% vol is equal to 10,000 ppm. The oxygen sensor reading starting point for normal oxygen is 20.8% vol or 20.9% vol, depending on the instrument manufacturer. Most oxygen sensors’ operating range are 0% vol to 30% vol in 0.1% vol increment changes. The Occupational Safety and Health Administration (OSHA) defines oxygen levels below 19.5% vol or above 23.5% vol as IDLH atmospheres. An oxygen-deficient atmosphere is a health hazard, and an oxygen-enriched atmosphere is a fire hazard.
Oxygen levels can be lowered by consumption or displacement. Consumption involves air being used up by combustion or other reactions that consume the air. Consumption can be caused by rusting metal, ripening fruits, drying paint or coatings, combustion, or bacterial activities.
Displacement can occur when a gas or vapor is introduced into an area that is not well-ventilated. A material displacing air could be flammable, toxic, corrosive, or inert (displaces oxygen). Carbon dioxide, nitrogen, and argon are examples of inert gases. Low oxygen levels will affect the operation of a catalytic bead sensor, the most commonly used sensor for flammable gas and vapor sensor. Oxygen-enriched atmospheres occur when an oxidizer is introduced into the air. An oxygen-enriched atmosphere increases the fire hazard of an environment by enhancing the ease of ignition and intensity of the combustion process.
If displacement is suspected, a decrease of 0.1% of the oxygen sensor reading indicates 5,000 ppm of the presence of a material-displacing oxygen. This concept can be simplified. We will use 20.9% oxygen and 79.1% nitrogen, argon, and trace gases to explain the displacement of oxygen. The introduction of 5,000 ppm of a material into air is equal to 0.5% vol of change. The material displaces 0.1% vol or 1,000 ppm of oxygen and 0.4% vol or 4,000 ppm of nitrogen, argon, and the other trace gases. This is known as the 1/5 rule. It is important that firefighters recognize that a decrease or an increase is a significant change and can present an increased risk.
Flammable Gas/Vapor Sensor Response Readings
The flammable gas and vapor sensor response readings operating range falls between 0% LEL and 100% LEL in 1% LEL increments. The response reading is relative to the calibration gas and the gas or vapor present in the atmosphere. OSHA defines 10% LEL as an IDLH atmosphere. A % LEL of 1 indicates that a flammable gas or vapor is present in an atmosphere and needs to be treated with respect and mitigated. A % LEL response reading of 1% LEL to 10% LEL is unsafe.
If a gas or vapor is positively identified, a correction or relative response factor can be used to correlate the instrument reading and quantify the identified gas or vapor. A correction or relative response factor is a multiplier that compensates for the difference between the response of the identified gas or vapor and the response of the calibration gas. Some manufacturers provide response or correction factors in their manuals, sensor specification sheets, or other supporting documentation. Use only correction factors specific to the manufacturer, sensor type, and instrument. Materials with a response or correction factor greater than 1 will have a lower detector response than the calibration gas. The response or correction factor can be calculated manually. Some instrument manufacturers allow for the selection from a database built into the instrument functions to automatically calculate the number. If an instrument has a built-in database, set the instrument to the specific calibration gas setting if the gas or vapor has not been positively identified.
Maintain and Train
Firefighters need to be trained in the operation and maintenance of the instruments. They need to understand sensor technology operating principles, limitations, and pros and cons. They need to understand the importance of using a calibrated and fully functioning instrument to ensure the validity of readings, and they must understand the how and why of routine calibration, fresh-air setup, and bump test.
As with fire behavior and fire suppression operations, firefighters need to understand chemical and physical properties and to train on incident investigation and response procedures where gas detection instruments are used. Lack of training could cause firefighters not to understand the instrument response and lead them to distrust the instruments and to disregard an instrument response. An instrument is only as effective as the experience and training of the firefighter and how well the instrument is maintained.
Bibliography
GFG Instrumentation, “What hazardous gases are associated with lead acid battery charging stations?” Retrieved from https://goodforgas.com/hazardous-gases-associated-lead-acid-battery-charging-stations/.
Handy, R.; Newell, D.; Dunn, B.; Yang, W. “Four-Gas Air Monitors: A Survey of Fire Responders’ Competencies,” Fire Engineering, November 2015, Volume 168, Number 11, pages 53-61. Retrieved from https://emberly.fireengineering.com/2015/12/03/291772/four-gas-air-monitors-a-survey-of-first-responders-competencies/.
Hawley, Chris. Hazardous Materials Sampling and Detection Devices. 3rd ed., Jones & Bartlett Learning, 2020.
Onuska, III J. “Don’t Rush Gas Detection Sampling,” ISHN Magazine, November 2018, pages 28-29. Retrieved from https://www.indsci.com/globalassets/documents/published-articles/2018/Dont-rush-gas-detection-sampling.pdf.
U.S. Consumer Products Safety Commission, “Responding to Residential Carbon Monoxide Incidents/” Retrieved from https://www.cpsc.gov/s3fs-public/coguide.pdf.
U.S. Department of Health and Human Services, “Chemical Suicides: The Risk to Emergency Responders.” Retrieved from https://chemm.nlm.nih.gov/chemicalsuicide.html.
Wagner, D. “The Dos and Don’ts of Atmospheric Sampling.” Retrieved from https://www.indsci.com/globalassets/documents/published-articles/2019/the-dos-and-donts-of-atmospheric-testing——occupational-health—safety.pdf.
Wang, Y. “Why Do You Need 10% Vol Oxygen To Operate Catalytic Bead Sensor?” Occupational Health and Safety Magazine, August 2018, pages 28-30. Retrieved from https://www.indsci.com/globalassets/documents/published-articles/ohs_lel_10_o2_ywang_aug18.pdf.
Jim Leer is an insurance senior loss control consultant and certified safety professional. He is a 32-year veteran of the fire service and a Pennsylvania local level state fire instructor. He is as a firefighter for the Somerset (PA) Volunteer Fire Department and hazardous material technician for the Somerset County (PA) Hazmat Team. He has degrees in A.S. fire science, B.S. safety management, and M.S. safety sciences.