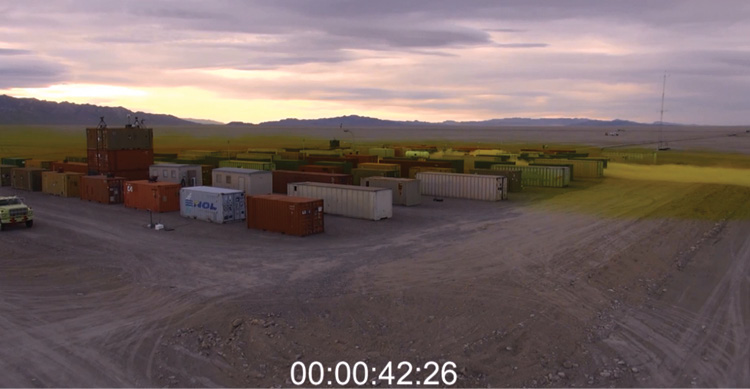
BY ANDREW BYRNES AND GREGORY G. NOLL
In “The Jack Rabbit Tests: Catastrophic Releases of Compressed Liquefied Gases” (Fire Engineering, November 2016, we introduced the Jack Rabbit Project and provided the history of the project and preliminary results of the 2015 and 2016 trials. The project’s final results and the potential impacts for emergency responders have been published in a report that can be accessed at the Jack Rabbit Project homepage at Utah Valley University (UVU) (https://www.uvu.edu/esa/jackrabbit/).
The “Jack Rabbit II Project” (JR II) was a joint effort that involved subject matter experts in hazardous materials and emergency response (the UVU Emergency Response Group) working collaboratively with scientists from the Department of Homeland Security—Chemical Security Analysis Center, the United States Fire Administration National Fire Academy, and the Lawrence Berkeley National Laboratory (LBNL) to ensure meaningful outcomes.
The JR II series of tests focused on catastrophic releases of chlorine, using the facilities of the U.S. Army’s Dugway (UT) Proving Grounds as the test platform. As part of the test team, the overall objective for the UVU Emergency Response Group was to answer questions for the emergency planning and response communities regarding planning for, tactical and operational considerations of, and public protective actions during a catastrophic chlorine release scenario. However, many of the conclusions could be applicable to any catastrophic release of a toxic inhalation hazard vapor or gas.
A “catastrophic” release differs from an accidental release where contents may be released over time caused by typical tank breach/release behaviors, such as those involving spills or leaks. In contrast, a catastrophic release scenario would result in the immediate and complete failure of the tank containment system, thereby releasing the contents immediately. An example would be an intentional act, such as one involving terrorism, which could represent a true “worst-case” scenario.
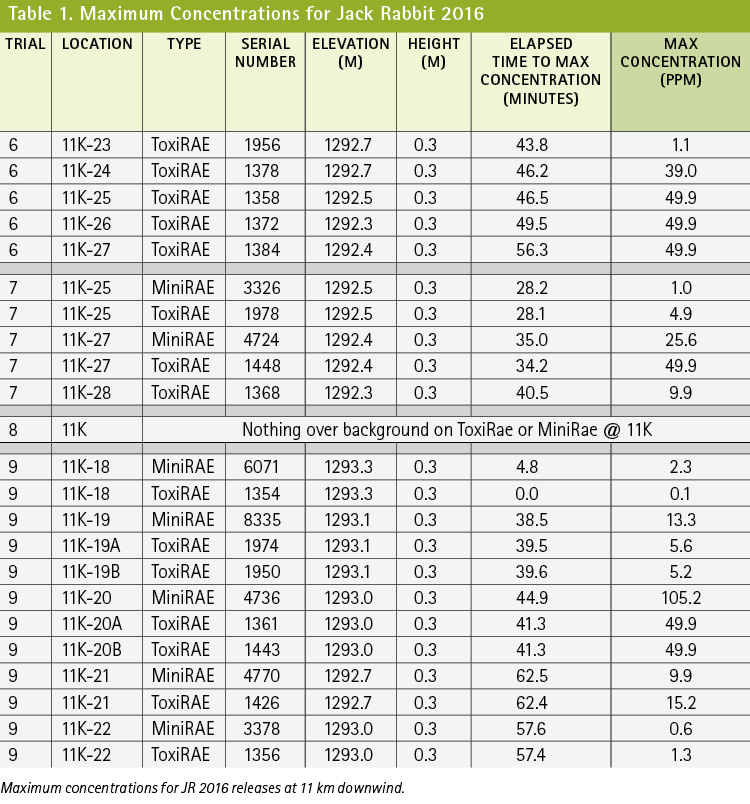
Conclusions Based on JR II Data
In August 2017, key contributors to the Jack Rabbit Project, including environmental systems researchers, plume modelers, emergency responders, regulators, and atmospheric scientists, were invited to UVU to formulate conclusions based on the JR II data. After expert presentations and extensive analysis of the data and a scientific consensus, the UVU Emergency Responder Group supports the stated conclusions to the following questions based on the JR II objectives as detailed in the final report.
Are the 2016 Emergency Response Guidebook (ERG) Initial Isolation and Public Protective Action distances consistent with the Jack Rabbit data in both the upwind and downwind environment?
Downwind guidance found in the 2016 ERG was found to be reliable and accurate to 11 kilometers (km) (7 miles) (Table 1). Note that variations in concentrations from detector to detector at the 11-km (7-mile) distance can be attributed to the naturally occurring turbulent boundaries of the plume and a characteristic nonuniform concentration of outdoor plumes attributed to air mixing and the distance from the release point.
Upwind guidance is a more complicated debate. Upwind but downhill conditions complicate the calculations and predictions for chlorine. The 2016 ERG indicates a 3,000-foot initial isolation distance (upwind distance) and a 7-mile (11-km) downwind protective action distance for a railcar release of chlorine at night in low-wind conditions.
Recommended protective actions for these release scenarios are usually sheltering in place (Table 2). Dr. David Brown with the Argonne National Laboratory stated that he “would not expect a fatality at 3,000 feet (ft.) [1,000 meters (m)] upwind”; however, “environmental and incident variables would indicate caution when deciding to move closer to the release.” Responders should use caution because of the many variables that affect a strict “upwind and uphill” policy. Response teams should further adjust the upwind distance based on an ongoing risk-based assessment process.

Brown considers “light-wind” or “low-wind” conditions to be < 2 miles per hour (mph). In very low wind conditions, the plume will not travel as far. As a result, concentration durations are longer and the plume width gets wider as one gets closer to the release point. Moderate winds of 5 to 7 mph move the plume through vulnerable populations with greater energy, making emergency response operations more difficult and subject to higher levels of risk. Higher wind speeds (more than 7 mph) will quickly move the plume away from the source where concentration is still a concern; however, plume duration and width are minimized closer to the release.
During the largest test release (Trial 9) of 20 tons of chlorine, readings were taken at 50m (165 ft.) and 100m (330 ft.) upwind and 0.3m (1 ft.) and 1.5m (5 ft.) above ground level (Table 3). Results showed concentrations at 0.3m of 2.8 parts per million (ppm) at 50m and 0.2 ppm at 100m. At first glance, this data would lead one to believe that the ERG is too conservative in the upwind environment. In defense of the ERG, Brown makes the point that because of so many unpredictable variables, including terrain, the direction of the jet release energy, and the potential channeling effects, “conservative isolation and protective action distances are intentional to protect vulnerable populations.” The UVU Emergency Responder Group supports this approach and the upwind distances found in the current ERG regarding rail tank car releases of chlorine.
Is sheltering in place the most survivable option as a primary means of public protection during such an emergency if evacuation is not possible? Is it better to be inside a structure or vehicle than outside until the outside chlorine concentration drops and the danger has passed?
Interior concentrations are greatly influenced by the peak level of the plume, which passes outside the vehicle or structure. If the peak concentration is high, there is a corresponding high concentration inside the vehicle/structure that must be exhausted from the interior over time. Dr. Mike Sohn of the LBNL explained that during the JR II experiments, the chlorine mixed evenly with the air inside both the vehicles and structures. This was typical of his experience with gas/vapor movement in other studies. He said, “Vapor density was less of a factor inside (a structure) due to natural air mixing.” Sohn then addressed mitigation strategies supported by the UVU Emergency Response Group and recommended the following public protective actions during a release:
- It is almost always better to stay inside than go outside.
- Where feasible, close exterior openings and shut down ventilation (heating, ventilation, and air-conditioning) systems.
- Retreating to an interior room without windows that is away from the exterior walls increases protection by an order of magnitude.
- Stay inside until the outdoor concentration is lower than the inside concentration.
There will come a time during a release scenario when the concentration outside the structure or vehicle will be less than the concentration inside. Inside a structure or vehicle, the lower concentration will have a longer duration than outdoors. Outside the structure or vehicle, the higher concentration will have a shorter duration (Figure 1).
When will it be safe to exit? It depends and is a variable timeline. “Wind is King” (i.e., wind speed and direction) when considering when and if to move outside.
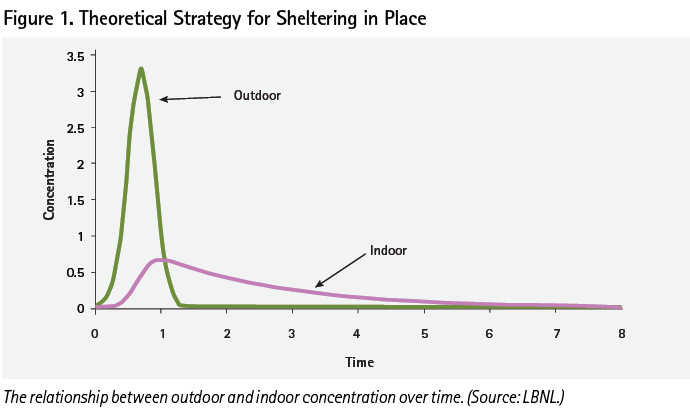
In summary, the duration of the high concentrations around a vehicle or structure will depend on the outside wind speeds, the absence of urban or natural barriers around the structure, and the amount of environmental sorption surfaces (e.g., trees, soil, and vegetation).

How are gas concentrations affected by environmental factors, primarily wind and terrain?
Wind and terrain are key factors in making risk-based tactical decisions about upwind positioning by emergency responders. In the 2015 JR II trials, barriers on the test grid appeared to influence greater upwind creep of the visible plume caused by channeling—the “canyon” effect—from the energy of the jet release moving between the Conex boxes. Much of the release energy in the 2015 JR II trials was deflected laterally (east and west) because of the configuration of the Conex boxes on the test grid (photo 1). In a response scenario, barriers such as solid fences, tree lines, buildings, and raised rail beds would certainly have a great influence on the upwind or lateral creep of the plume. Shifting winds and other environmental factors would also make the upwind environment less predictable.
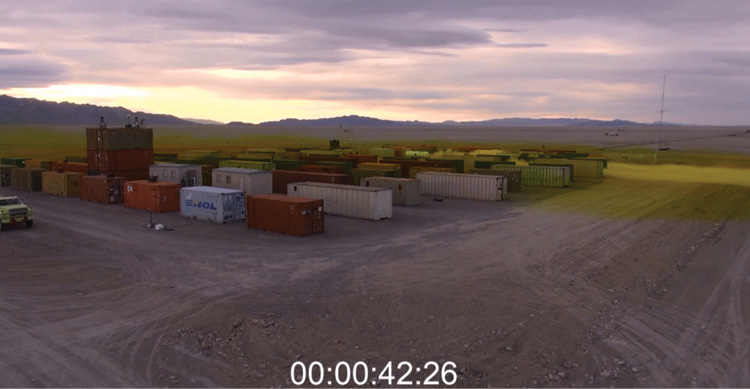
(1) JR II Trial 5, 2015. This is a diagonal view from the northwest corner looking southeast toward the chlorine container release. Wind direction was almost directly centerline from the south at 182° and 2.7 m/sec (~6 mph). This view shows the inclination for the plume to move lateral to the wind. (Photo courtesy of Department of Homeland Security—Chemical Security Analysis Center.)
Will vehicles continue to be operational even when exposed to ultra-high concentrations of chlorine?
The JR II trials in 2015 and 2016 confirmed an increasing probability that gasoline and diesel engines will likely continue to run in ultra-high concentrations of chlorine (240,000 ppm = 24 percent volume of gas in air in Trial 5, 2015). If emergency responders find themselves in a position of danger inside a vehicle, they should move laterally to the wind and the plume at the highest rate of speed that considers safety. The engine of the vehicle, whether gasoline or diesel, should continue to run. Although the JR II trials did not test either engine type under load (high rpm), they did remain running at idle, which presents the probability that they will remain operational. Responders should roll up windows, shut down vehicle ventilation/fan systems, and don self-contained breathing apparatus if possible. Move fast, act fast, and retreat to an area of safety. Experience with real-world accidental releases (Macdona, Texas, chlorine and Minot, North Dakota, anhydrous ammonia) have shown that getting out of a vehicle or structure and attempting to exit the area on foot downwind of the release is not a safe alternative. Once through the plume, all windows should be rolled down completely and the vehicle ventilated with vent systems turned up to high speed. This takes the contaminated inside air from 1.6 air changes per hour to more than 125 air changes per hour in seconds as seen during the JR II vehicle experiments per Dr. Woody Delp, LBNL.
Will photoionization detectors (PID) with 11.7 eV lamps detect chlorine with reasonable accuracy and repeatability over broad chlorine concentration ranges?
During the JR II trials in 2016, the UVU Emergency Response Group used a PID for detection and monitoring activities. Chlorine has an ionization potential (IP) of 11.48 eV. The JR test series showed that PID technology with an 11.7eV lamp was able to detect chlorine in real time with reasonable accuracy and repeatability in broad chlorine concentration ranges. However, it could not be detected when using the more commonly carried 10.6 eV bulb. The manufacturer of the PID recommended changes to its calibration gases and response factors referencing data from JR II.
There are significant data to support the application and limitations of PIDs with 11.7 eV bulbs when exposed to various concentrations of chlorine. Emergency responders should be assured that PID technology, when using an 11.7 eV lamp and properly maintaining it after repeated exposures to high concentrations of chlorine, is reliable and will continue to recalibrate and provide useful results.
What are the capabilities and limitations of common plume dispersion models like ALOHA®, and what is the proper application of these models in real time?
The primary strength of predictive plume models is in their use as planning guidance or forecasting tools rather than as emergency response tools because of the real-time uncertainty of some essential source data. Given the known inputs provided by the JR II trials, ALOHA® (Area Locations of Hazardous Atmospheres) was consistent in the way it depicted the output of the model. However, the inherent limitations of single-point atmospheric data input to the model does not allow for adjustments of the plume calculations as it moves downwind over long distances.
As seen in JR II Trials 1-5 in 2015, barriers in the path of the plume had a significant effect on both upwind and downwind plume behavior. ALOHA® does not account for these steering effects and meanderings within its 10-km range. The user needs to be cognizant of the limitations of the model and how it depicts the output. Trials 6-9 in JR II 2016 were unabated over flat terrain for which the model would theoretically be more accurate. Data analysis from these releases leads to the conclusion that the ALOHA® model is qualitatively sound.
The reality of an incident is that emergency responders will not have the luxury of collecting release point parameters in a timely manner, thereby putting any model output in doubt when it comes to real-time, on-scene plume predictions. It should be emphasized that this issue is not exclusive to ALOHA®. Container source data inputs (e.g., flow rate, size of the breach/opening, and type of release) will determine the accuracy of the model output. Experience shows that this will be the most difficult information for responders to collect and input into the plume model.
Many variables that are unknown to the responder running the model may make the ALOHA® output questionable for on-scene incident management purposes. Again, source data is critical. The model is only as good as the source data you have. The UVU Emergency Response Group advises caution in making tactical decisions based on a plume model where the source data is questionable. The Final Jack Rabbit Report, available on the UVU Jack Rabbit Web site, goes into further detail on this subject.
What is the effect of chlorine, an oxidizer, on common urban surfaces and the equipment used in emergency response?
Common urban surfaces and materials were not greatly affected, even by direct exposure to liquid chlorine. No residual chlorine contamination was noted on any of the surfaces, although discoloration was common. Heavy hydrocarbons dissolved and metal surfaces were immediately corroded (photo 2). Electronics continued to operate after exposure; however, long-term operability was erratic. Copper and bare steel seemed to be the two most affected metals showing immediate and aggressive corrosion. Observations assured the UVU Emergency Response Group that common urban materials were unlikely to burst into flames.
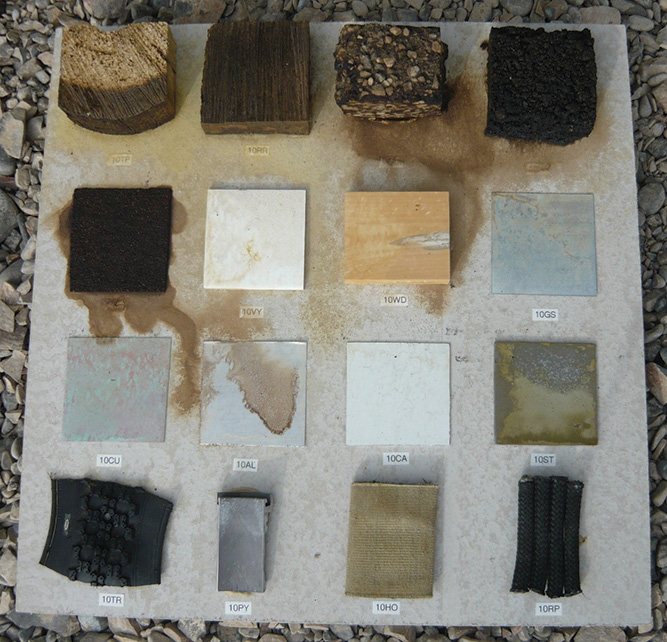
(2) Trial 5, 2015. Witness board #10 after complete evaporation of the liquid chlorine exposure. Note the dissolving hydrocarbons and metal corrosion. (Photo by Andrew Byrnes.)
Can a risk-based response process be effectively used during incidents involving catastrophic releases of TIH vapors or gases? How will the aerial video of the plume affect a risk-based decision process?
The UVU Emergency Response Group found that the application and use of a risk-based response process is critical to the delivery of a safe and effective emergency response. Input factors should consider container design and construction, container stress/breach release behavior, wind, terrain, exposures, and other environmental conditions. The Jack Rabbit Project was impactful for the emergency planning and response communities in that it provided an objective platform for the scientific validation of long-practiced and accepted hazmat strategies and tactics.
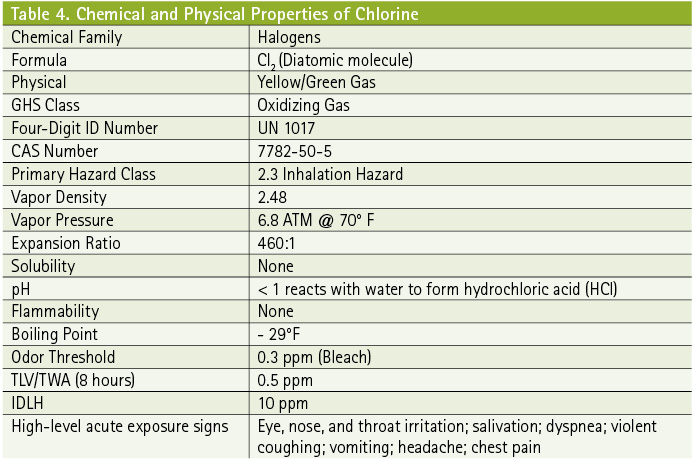
Emergency responders will likely not change the way they tactically approach a catastrophic chlorine release based on the JR II data, which includes aerial video of plume behavior. However, the observations in the video files related to the speed, the lifting behavior, the visual aspects, and the density of the chlorine plume are key elements in assessing plume behavior and applying risk-based response processes, especially as they pertain to the evaluation and selection of public protective actions. Emergency responders will gain an appreciation for the potential speed and scope of a release when they view the videos on the UVU Jack Rabbit Web site. Emergency responders should continue to base their incident decision making on the facts, science, and circumstances of the incident. The Jack Rabbit Project has successfully added to the body of understanding of the facts and science of this process, given certain test conditions and climatic circumstances.
The Risk-Based Response Based on NFPA 472
Authors’ note: We recognize those individuals involved in the Jack Rabbit Project and the successful production of the final data and outcomes: David Matthew, independent fire researcher, Kansas; Hank Dupont, Kansas Office of the State Marshal-Hazmat Emergency Response; Wayne Yoder, hazmat training program manager, National Fire Academy, Emmitsburg, MD; Christina Baxter, Ph.D., Emergency Response Tips, LLC, Woodbridge, VA; Woody Delp, Ph.D., Lawrence Berkeley Labs, University of California at Berkeley; Mike Sohn, Ph.D., Lawrence Berkeley Labs, University of California at Berkeley; Mark Whitmire, U.S. Army Aberdeen Proving Ground, MD; Col. Ronald F. Fizer, former commander, U.S. Army Dugway Proving Ground, UT; Damon Nicholson, Dugway Proving Ground-Test Management Branch, UT; Chief Patrick Carnahan, Dugway Fire & Rescue Department, UT; Kelly Wright, formerly of the U.S. Army Dugway Proving Ground, UT; and Shannon Fox, Ph.D., U.S. Department of Homeland Security—Chemical Security Analysis Center.
Reference
Noll, Gregory G and Byrnes, A. (November 2016) “The Jack Rabbit Tests: Catastrophic Releases of Compressed Liquefied Gases.” Fire Engineering. https://bit.ly/2UGRDi5.
ANDREW BYRNES, TASC-F, EFO, M.Ed., is an associate professor with the Utah Valley University (UVU) Emergency Services Department in Provo, Utah, and the director of the UVU Firefighter Recruit Candidate Academy. He retired as the special operations battalion chief with the Orem (UT) Fire Department. Byrnes served as a law enforcement officer for 18 years and a paramedic for 16 years. He is an emergency services instructor and course reviewer and has instructed at the Center for Domestic Preparedness and the National Fire Academy.
GREGORY G. NOLL, CSP, CEM, is the senior planning specialist and retired program manager for the South Central Pennsylvania Regional Task Force. He has 48 years of fire/emergency response service, including 29 years with the U.S. Air Force—Fire and Emergency Services. The co-author of nine textbooks on hazardous materials emergency response and management, he is a member and past chair of the National Fire Protection Association Technical Committee on Hazardous Materials/Weapons of Mass Destruction Response Personnel. He also serves on the International Association of Fire Chiefs Hazardous Materials Committee, the Fire Engineering Editorial Advisory Board, and the InterAgency Board for Emergency Preparedness and Response—Training and Exercise SubGroup.