By ANDREW BYRNES
The National Fire Academy (NFA) recently launched an exciting new course, Management and Safety in Response to Hazardous Materials/WMD Incidents; I was fortunate enough to be part of its course development team. In the process of building out scenarios for the class, I suggested using a liquid oxygen (LOx) spill on a freeway with the standard precautions that would follow this type of release.
- Exploding Liquid Oxygen Tanks Shuts Down Seattle (WA) Highways
- CRYOGENIC LIQUID OXYGEN CONTAINER EXPLOSION: AN INVESTIGATION
- How You Will Have Your Oxygen: Gaseous, Liquid or Solid State
Discussing lessons learned when dealing with LOx, we identified the following “standard” precautions necessary to ensure responder safety:
- Do not step on the frozen asphalt; it could explode.
- Do not drive over the frozen asphalt; the pressure could detonate under the weight of the vehicle.
- Do not allow LOx to contact combustible materials such as dried grass in the median or hydrocarbons such as diesel fuel because it will spontaneously ignite, resulting in a fast and intense fire.
- Wait 30 minutes after the last frost is gone before stepping on the asphalt.
These precautions were based on traditional guidance and commonly accepted as accurate. As experienced hazmat instructors, we had all taught these precautions over the years. However, those of us with hazmat chemistry backgrounds were skeptical. None of us had really asked ourselves if these precautions had ever been scientifically tested and verified.
Understanding that oxygen (O2) is a powerful oxidizer, I asked myself, would it really “ignite” hydrocarbons by simply coming in contact with them? Would the activation energy be sufficient to initiate combustion? Without testing theories, we were guessing and then passing on our best guess as hazmat training. I decided it was time to test the “standard” precautions related to LOx response. So, we passed on using a LOx scenario for the new class.
Searching for the Answer
Being at the NFA, I had the National Emergency Training Center Library as a tremendous resource to find the answer to my question. I spent hours researching the books, articles, and research papers that were in any way related to LOx. The sum of the precautions and “facts” I found regarding LOx were unsubstantiated and not independently verifiable. Most of the research was anecdotal and similar to Martel’s Chemical Risk Analysis, “George Claude was seriously injured in 1903 after inserting a candle into liquid oxygen,” or from National Fire Protection Association (NFPA) 53, Recommended Practice on Materials, Equipment, and Systems Used in Oxygen-Enriched Atmospheres, Annex D, which lists many types of LOx incidents but with the caveat, “NFPA cannot guarantee the accuracy of the reports” (NFPA 53, D.1.3). None of the 63 “incidents” in NFPA 53, Annex D, were corroborated. Several vendors have produced safety data sheets that state vague cautions such as LOx will violently oxidize organic material. On the handling and use of LOx systems in health care facilities, the Compressed Gas Association pamphlet 2.7 states, “Stepping on or rolling equipment across a liquid oxygen spill can result in explosive ignition of combustibles” (CGA, 4.1.2.7).
I asked the students in my class at the NFA and members of the technical committee for NFPA 470, Hazardous Materials/Weapons of Mass Destruction (WMD) Standard for Responders, if they had ever heard of any “explosions” when coming in contact with LOx. In my conversations, there were no experiences with ignition caused by pressure or any witnessed hypergolic reactions with LOx and combustibles. In my research efforts, I could not find a verifiable incident anywhere in the nation.
Regardless, our testing at Utah Valley University (UVU) showed that, when LOx saturates a combustible material and then an ignition source is introduced, violent and vigorous combustion occurs with increased burn rates, light, and heat. My review of the literature in fire service publications indicates that LOx spills have proven dangerous in the presence of an ignition source. Without an ignition source or when ignition sources were controlled, LOx did not pose a significant hazard beyond those associated with cryogenic liquids.
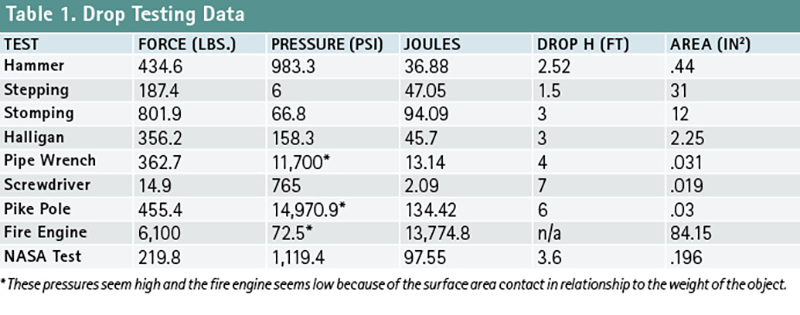
Our goal at UVU was to conduct field trials in a practical application to verify the aforementioned “dangers” of LOx. We conditioned several asphalt samples roughly 3½ inches square and three inches thick under LOx for a period of 30 minutes. We then subjected the frozen asphalt samples to a series of impact tests by raising the object to a specified height and dropping it onto the sample. The first two were a step and a “stomp” in a weighted rubber fire boot. We then struck samples with a 10-pound sledgehammer; dropped a halligan (blunt head first), pipe wrench (head first), pike pole (point first), and screwdriver (point first); and drove a fire engine over a larger-conditioned asphalt surface and liquid pool of LOx. Forces were determined by subjecting a force plate device to these objects and digitally measuring the results of the force and calculating the height, pressure, and energy of the drop (Table 1). None of the sources of mechanical impact or pressure caused any reaction in the asphalt after a minimum of five tests.
In 1970, the Apollo 1 capsule fire killed astronauts Gus Grissom, Ed White, and Roger Chaffe. They died in a near 100% O2-enhanced atmosphere when a fire broke out and consumed them before NASA could effect rescue. NASA became keenly aware of the dangers of LOx. To better understand liquid and gaseous oxygen environments, field testing of LOx-soaked asphalt and mechanical impact was designed and conducted by NASA in 1973 on runway materials. To everyone’s surprise, mechanical impact on LOx-soaked asphalt detonated and blew the apparatus NASA designed 30 meters into the air and created a debris field 50 meters in diameter.
The Tests
At UVU, we wanted to replicate and verify NASA’s 1973 test using a scientific method. We were successful in replicating the NASA test and so could endorse and validate the results. Because we were able to replicate energetic reactions using our apparatus, the results of the UVU tests would also have validity.
In 2017, the American Society of Testing and Materials (ASTM) created the Standard Test Method for Determining Ignition Sensitivity of Materials to Mechanical Impact in Ambient Liquid Oxygen and Pressurized Liquid and Gaseous Oxygen Environments (G86-17). ASTM codified the configuration of drop mechanisms used to test the sensitivity of materials to mechanical impact pressure. The apparatus designed and built at UVU was designed in compliance with the ASTM impact testing configuration (photo 1).
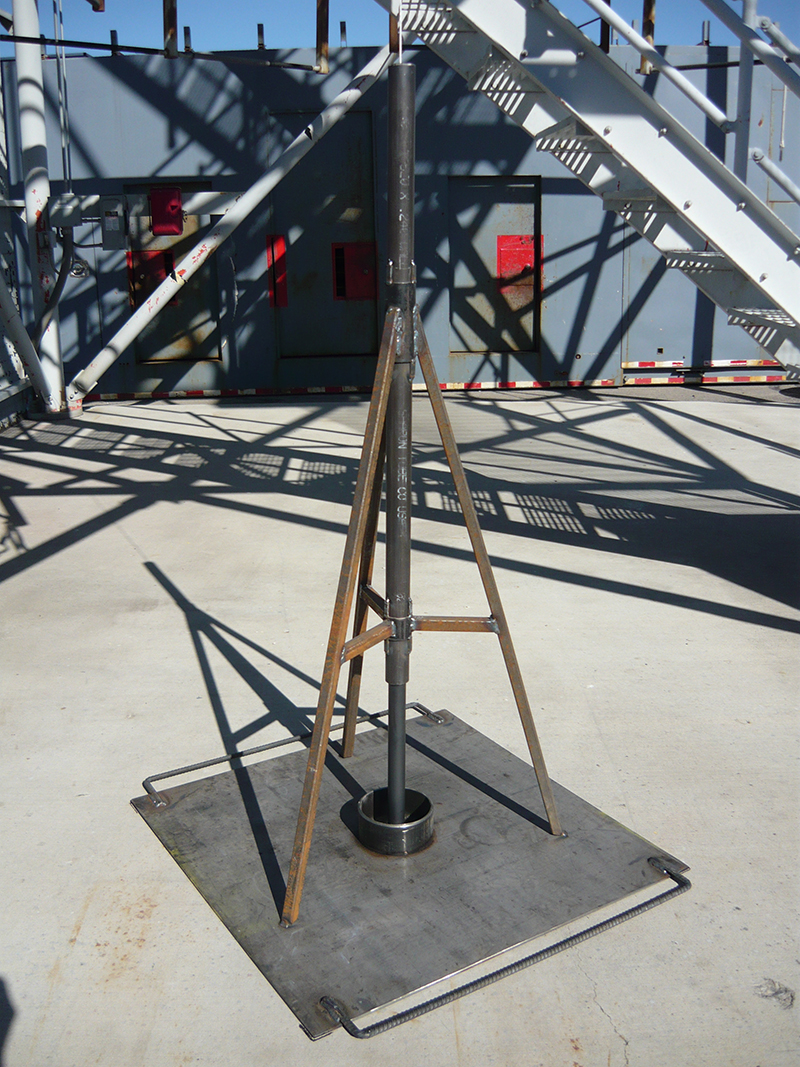
(1) The UVU test apparatus replicating NASA and ASTM specifications. (Photo by Eugene Ngai.)
ASTM G86-17 states that any one reaction in 20 drops of the mechanism (5%) indicates the tested material is “reactive.” We experienced five reactions in 20 drops of the plummet (25%) and were able to duplicate those reactions again 60 days later (photo 2). The tested strata were composed of crumbled asphalt, on which an aluminum block was placed, then covered by additional crumbled asphalt before being immersed in LOx (Figure 1). It was this configuration that exploded during the NASA test in 1973.
Figure 1. NASA Configuration of the Asphalt and Aluminum Strata
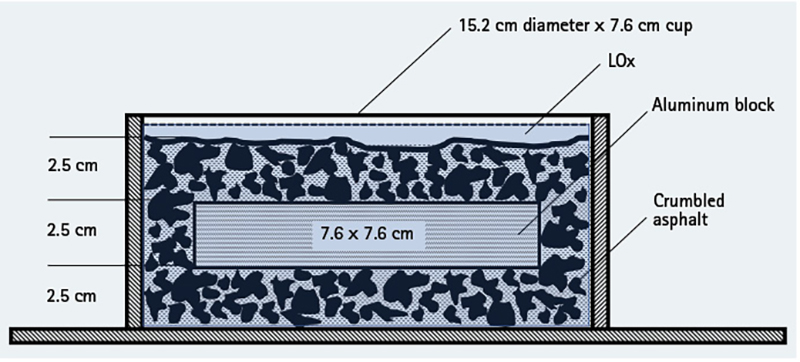
Figure by author.
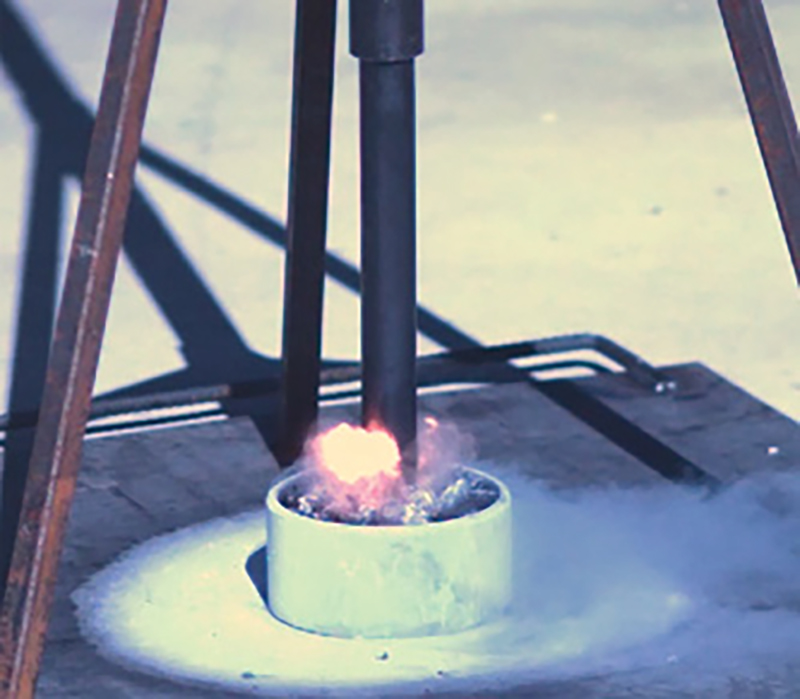
(2) October 21, 2021, UVU Test #16 reaction. (Photo by Brian Patchett.)
Dan DeMille and Brian Patchett at UVU captured the reactions using three high-speed cameras, one of which was used to capture real-time video and another a high-speed infrared thermal camera that was used to detect any thermal changes. These provided outstanding resolution and captured what the naked eye couldn’t see because of the speed of the reaction. In real time, we knew that a reaction had occurred when we heard what sounded like a gunshot. These audible reactions were heard by the research team and captured on video. However, sound-level data was not captured using audio-measuring devices.
From these tests, we concluded that mechanical impact could occur with the NASA configuration. However, after testing several control configurations, we found that a reaction would only occur using that unique strata. Our highways do not consist of crumbled asphalt covered with a one-inch-thick plate of aluminum, which is then covered with more crumbled asphalt; these reactions should be viewed in that important context. No reaction occurred with solid asphalt blocks and LOx, crumbled asphalt and LOx, LOx alone, or an aluminum block and LOx. The aluminum was the surface to which the microscopic bubbles in the LOx were compressed by the 20-pound plummet falling 43.3 inches when struck by the ½-inch-diameter stainless-steel striking pin.
Chemistry and physics faculty at UVU, Dr. Merrill Halling, and Patchett, in consultation with compressed gas expert Eugene Ngai, explained to me the likely ignition source. The reaction occurred as the plummet compressed the microbubbles in the LOx against a rigid boundary (an aluminum block), almost instantaneously increasing the pressure and, thus, the temperature of the O2 gas inside the bubble, then releasing that energy as adiabatic heat. Adiabatic heat differs from isothermal heat in that it cannot be dissipated. The pressure and heat serve as the ignition source for the hydrocarbons in the asphalt and the surrounding super-charged O2 environment. The adiabatic heat principle is similar to what occurs in the cylinder of a diesel engine, causing ignition through compression.
Interestingly, we subjected a used, soot-contaminated, leather firefighting glove and a brand-new glove with liners, both compliant with NFPA 1971, Standard on Protective Ensembles for Structural Fire Fighting and Proximity Fire Fighting, to the hammer test under LOx. The new leather glove had no reactions when struck six times. The contaminated glove had four reactions when struck eight times with the hammer—a 50% reactivity rate. Additionally, the contaminated leather was visibly damaged, and a loud report was heard with each of the positive reactions (photo 3). The new leather was completely intact after the tests.
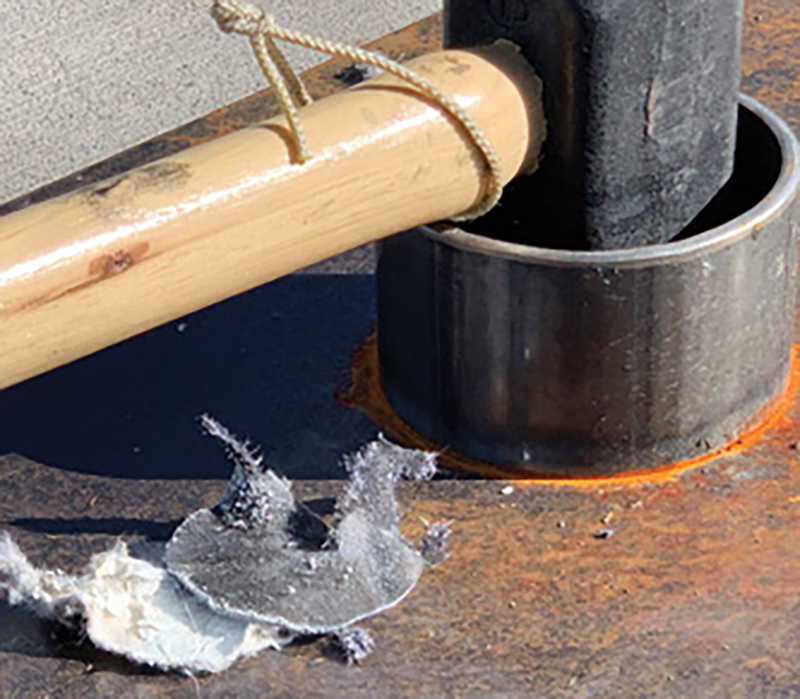
(3) The contaminated glove and the hammer test device. (Photo by Oscar DeMille.)
To determine nonimpact reactivity, we poured LOx directly onto an asphalt surface and dropped a road flare into the LOx pool. Other than some increased flare length and burning of the flare paper, no flaming combustion or explosion occurred. We poured LOx directly into 11 different hydrocarbon compounds commonly available and observed no reaction other than creating a frozen liquid. Saturated and unsaturated hydrocarbons, synthetic and natural compounds, alcohol-based products, various viscosities, and three liquids with flash points below 100°F did not react in contact with LOx. Likewise, combustible materials such as a cup of potato chips—oily and organic—did not react in contact with LOx. This having been said, when any of these combinations of hydrocarbon liquids or organic materials and LOx met with an ignition source, the combustion was rapid and intense. Combustion, influenced by LOx, is noticeably more rapid and vigorous than “normal” combustion occurring in our atmosphere of 21% O2.
The UVU tests also considered static electricity. We found a static spark to be an unreliable source of ignition. The spark was certainly hot enough (+1,800°F), but the duration of the heat source, only milliseconds, may have been too brief to cause ignition. Arcing, caused by shorting out the positive and negative sides of a 12V battery, produced visible sparks and molten metal beads, which immediately ignited any combustible fuel in LOx. Don’t discount the arcing of a vehicle battery short circuit on the scene when controlling ignition sources.
Based on these tests, we came to the following conclusions:
- LOx, soaked and frosted over asphalt, will not react from the pressure associated with being stepped or stomped on, driven over, or impacted by common response tools that are dropped on it or the pressure from a direct sledgehammer strike.
- LOx, spilled on asphalt, would be extremely difficult to ignite with common ignition sources found on the emergency scene. Heat sources added to LOx/asphalt combinations only increased the rate of vaporization of the LOx.
- LOx will not react on contact with common combustibles, organic materials, flammable materials or liquids, and other common hydrocarbons unless an ignition source is introduced, in which case the combustion will be violent and instantaneous.
- The NASA explosion from the plummet test in 1973 was successfully replicated at UVU. However, circumstances leading to the detonation of the LOx and asphalt configuration are unrealistic—i.e., the aluminum plate inserted in a crumbled asphalt stratum. Explosions could not be replicated using solid or crumbled asphalt and LOx alone, a much more likely configuration.
- Practical testing of hazards associated with LOx should be expanded in the future.
- Responders should take every precaution necessary when dealing with the primary hazards of LOx, namely embrittlement of surfaces in contact with the super-cooled liquid, high expansion ratios, and frost formations. Anytime LOx is mingled with combustible materials or flammable and combustible liquids, sources of ignition should be eliminated because of the possibility of extremely vigorous combustion.
Any modifications to your agency’s procedures should be evaluated carefully based on these conclusions.
References
American Society of Testing and Materials. (2017). Standard Test Method for Determining Ignition Sensitivity of Materials to Mechanical Impact in Ambient Liquid Oxygen and Pressurized Liquid and Gaseous Oxygen Environments (G86-17). ASTM International.
Compressed Gas Association. (2018). Guideline for the safe storage, handling, and use of small portable liquid oxygen systems in health care facilities. 5th Ed. P-2.7-2018. CGA Inc.
Martel B. (2000). Chemical risk analysis: A practical handbook. London: Penton Press.
Moyers C, C Bryan, and B Lockhart. (1973, December). Test of LOX compatibility for asphalt and concrete runway materials. TM X-64086. National Aeronautics and Space Administration (NASA). Kennedy Space Center, FL.
National Fire Protection Association. (2021). NFPA 53, Recommended practice on materials, equipment, and systems used in oxygen-enriched atmospheres. NFPA. Quincy, MA.
ANDREW BYRNES, M.Ed. EFO, retired as a special operations battalion chief from the Orem (UT) Fire Department. He was a sworn law enforcement officer for 18 years and a paramedic for 16 years. Byrnes is also a professor in the Emergency Services Department and the director of the Firefighter Recruit Candidate Academy program at Utah Valley University. He is a course designer and instructor for the National Fire Academy, specializing in hazmat/weapons of mass destruction (WMD) chemistry and advanced science, and is a graduate of its Executive Fire Officer Program. Byrnes has reviewed and contributed to several textbooks related to hazmat/WMD chemistry and response and is a frequent course reviewer, researcher, and subject matter expert in the areas of hazmat, firefighting, leadership, and management. He is a member of the NFPA 470 Technical Committee. He has an associate degree in fire science, a bachelor’s degree in public emergency services management, and a master’s degree in education from Utah State University.