BY RICHARD C. BEAULIEU
The nation’s first-due companies are responsible for the beginning stages of any hazardous materials emergency, whether at a residence, at a chemical facility, on a roadway, at an airport, at sea, or on a railroad. Hazmats are used everywhere; while they have made modern life possible, they pose a significant risk to human health when released from their containers.
- Hazmat Size-Up: A New Model for Rapid Decision-Making
- Hazmat Metering for First-Due Companies
- Culture Change: Viewing Extinguished Building Fires as Hazmat Scenes
- RAPID HazMat for Safe and Quick Response
Aside from the few hazmats that require additional research because they break accepted rules, they have physical and chemical properties that make their behavior predictable. Understanding these properties and how they relate to the predictability of a given compound is paramount for first responders’ safety. When lives are at stake, first-due companies will not always have knowledgeable hazmat technicians present and must take actions and make decisions well before a hazmat response team responds.
Solids, Liquids, Gases
Any hazmat could be dangerous to responders, especially in conditions where chemical reactions could take place. Therefore, in whatever physical state the matter is in—solid, liquid, or gas—responders must take it seriously until they have gathered information about it.
Solids generally pose the least risk since they have a definite mass, a definite volume, and a definite shape. They are easy to see and are unlikely to extend too far beyond the source of the release.
Liquids pose a greater risk since they have a definite mass and definite volume but no definite shape. They conform to the area of release based on gravity and, depending on their viscosity, may flow away from the area of origin and enlarge the contaminated area.
Gases pose the greatest risk; they have a definite mass but no definite volume or shape, can engulf the entire area of origin, and have a wide array of general hazards. Gases can be colorless, odorless, and tasteless or all three at the same time, as is carbon monoxide, which is well known in the fire service. Natural gas is as dangerous as it is abundant in daily life, and so this colorless, tasteless, and odorless gas has an odorant added to it for safety. Gases can be toxic, corrosive, carcinogenic, and flammable/explosive. They can violently support combustion, react with other substances readily, displace oxygen (as a simple asphyxiant), and directly disrupt the body’s ability to oxygenate tissue (as a chemical asphyxiant). Finally, pressure and energy are among the greatest dangers that gases present. In transportation, in storage, and as used in various processes, gases are under pressure. Pressure in the hazmat equation increases risk significantly. Firefighters must understand the physical and chemical properties of gases to recognize the dangers of a given incident and enhance their risk/benefit analysis to keep all responders safe.
Physical Properties
A material’s physical properties dictate how it will behave—e.g., how temperature and pressure can transform a compound into a different state of matter without changing the compound chemically. Depending on the weather or the ambient temperature of an indoor facility, a chemical released from its container will act predictably. On release, gases are subject to atmospheric pressure, which is 14.7 pounds per square inch (psi) or 760 millimeters of mercury (mmHg).
Temperature and pressure are used to convert a gas into a liquid to enable shipping as much of the product as possible to the facilities that ordered the compound for their processes. Firefighters who understand physical properties and how industry uses these properties are better equipped to make competent decisions at a hazmat incident. Remember the simple rule that in a closed container, as temperature increases, pressure increases; conversely, as temperature decreases, pressure decreases. The faster something loses pressure, the faster it becomes cold; the faster something is pressurized, the faster its temperature rises. For example, think how warm a self-contained breathing apparatus bottle becomes when it is filled too quickly or how cold the valve becomes when the bottle is opened and allowed to rapidly vent.
Boiling Point
The boiling point is the temperature at which a liquid can be converted to a gas. The value is different for every compound but can be learned through research; below this temperature, the compound will be in the liquid phase. Another definition of boiling point is the temperature at which vapor pressure equals atmospheric pressure. All liquids have a given vapor pressure (the pressure at the surface of a liquid) at a given temperature until they reach their boiling point. These properties can be researched, and reference materials will provide data for the material at a standard temperature range of 68°F to 77°F.
For example, acetone has a vapor pressure of 180 mmHg at 68°F (room temperature). Water at the same temperature has a vapor pressure of roughly 25 mmHg. Acetone will not reach 760 mmHg (atmospheric pressure) until 133°F (its boiling point). Although this article is about gases, it is important to know that when researching a liquid that has been spilled, the higher its listed vapor pressure on the reference materials, the more vapors it is readily giving off, especially if the ambient conditions are much warmer than room temperature. These vapors can be as toxic, as corrosive, as flammable, and as carcinogenic as any gas encountered.
When researching the boiling point, if the number is negative, then it is safe to assume that almost anywhere in the country, if it is released from its container, that compound will be a gas. Anhydrous ammonia has a boiling point of -28°F; not many places in the continental United States will see a temperature below that in a given year. However, hydrogen cyanide has a boiling point of 78°F; therefore, depending on the time of year, a release of this product from its container could be in the liquid or the gas state.
Vapor Density
Vapor density is the density of a gas relative to the density of air. This number is based on the molecular weight of air, which is 29. Molecular weight is a number found under each element on the periodic table for each atom of that element. Therefore, the molecular weight of a compound can be found by adding up the molecular weights of all the atoms present. Any gas with a molecular weight below 29 will rise, and any gas with a molecular weight above 29 will sink. Reference materials may call this relative gas density (RgasD) or vapor density. Air has a RgasD of 1, so any value that is >1 means that the gas will sink; any value <1 means that the gas will rise.
Anhydrous ammonia has a RgasD of 0.6, so it is lighter than air and will rise. Chlorine’s RgasD is 2.47, so it is heavier than air and will sink and settle into low areas. This is important when considering whether to shelter in place, understanding that where the gas is going to go is crucial for explaining to people where they should shelter in their homes. Any large release of a flammable/explosive gas is cause for evacuation of the area closest to the release because it is impossible to control the ignition sources in every home affected by the release. It is also important to know that most gases sink. There is only a short list of chemicals that are lighter than air; these are contained in the mnemonic 4H-MEDIC-ANNA (Table 1).
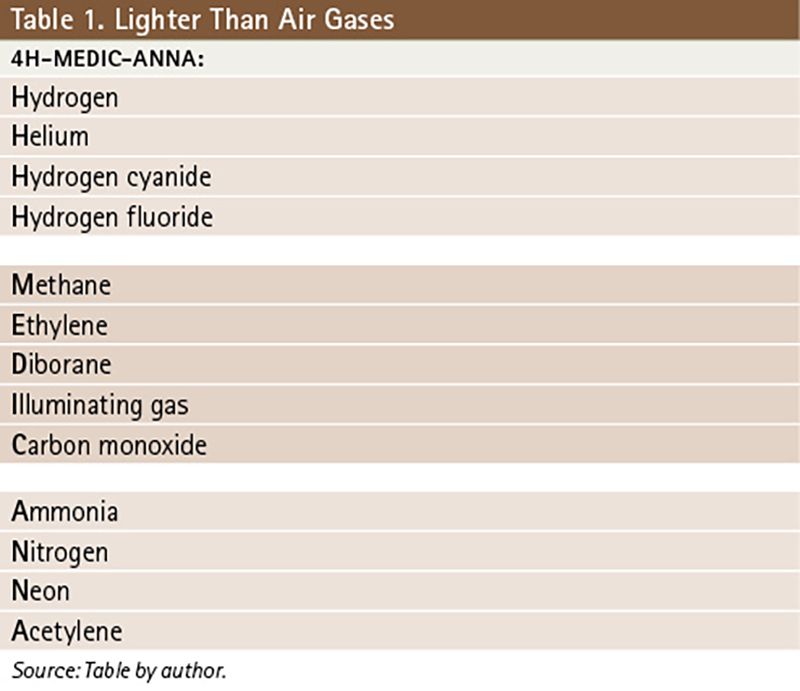
Industrial Processing of Gases
Based on the physical properties of a gas, an industry will process, transport, or store gases based on the end user’s requirements. The goal is to ship or have as much product on site as possible to minimize the need for excessive transport and transfer of gases.
Oxygen is a good example. In the back of an ambulance, a cylinder of compressed oxygen (a house cylinder, which varies in size) is used to treat patients and will last about a week or two, depending on the call volume and size of the cylinder. Hospitals use large volumes of oxygen daily and so have liquid oxygen tanks on the hospital campus providing them with several weeks’ worth of oxygen. Cylinder gases are classified as compressed, liquefied, or cryogenic.
Compressed Gas
Compressed gas remains solely in the gas phase in a cylinder but it is pressurized to fit as much gas as possible by forcing the gas molecules together. Cylinder failure is the greatest danger with these cylinders since their contents are under pressure, so a cylinder or valve failure could cause injury from the product itself or the trauma of an explosion and resulting projectiles. These cylinders are also subject to fail under fire conditions and become projectiles as the pressure in the tank exceeds the rating of the steel or valve assembly. Common daily examples of compressed gases include oxygen in medical settings or residences, helium at stores or residences, and acetylene at workshops or garages.
Liquefied Gas
Liquefied gases exist as both a liquid and a gas in a cylinder. Industry applies a certain pressure to a given gas to force its molecules to pack together so tightly that the gas is forced into the liquid phase, allowing them to put a great deal of product in the cylinder for shipping and distribution or for on-site use at a facility. The expansion ratio is the reason for this.
For example, if the propane in a cylinder used for an outdoor grill was only compressed, it would need to be filled every other cookout. However, because the propane is liquefied, one part of liquid is equivalent to 270 parts of gas, which is the expansion ratio, expressed as 1:270. Even though the boiling point of propane is -45°F, the pressure applied to the gas keeps it a liquid regardless of the temperature outside.
When companies fill a liquefied gas container, whether the tank is as small as a propane cylinder or as large as a railcar, the maximum liquid fill will be 80 percent of the cylinder to allow for expansion of the liquid during warmer days; the space left over will be occupied by the gas phase of the compound—often called the vapor space. If a cylinder is overfilled, the expansion of the liquid product can cause tank failure. Typical liquefied gases seen in the field include liquefied petroleum gas, anhydrous ammonia, hydrogen chloride, and chlorine.
The key to safety with liquefied gases is understanding the physics of what happens on release from their cylinder and to always remember that anything under pressure greatly increases risk. Liquefied gases have very low boiling points; propane’s is -45°F and anhydrous ammonia’s is -28°F. These products could be in fixed-facility tanks for various processes (anhydrous ammonia is used in refrigeration) or they could be found in transportation in any size from a 20- to 150-pound cylinder to a roadway or railcar tanker. Even though the liquefied gas in its container will be at the current temperature it is exposed to, if the tank is punctured at the liquid level, the pressure of the leaking liquid will rapidly decrease to atmospheric pressure, which rapidly decreases the substance to its boiling-point temperature, which is cold enough to cause instant frostbite injuries. The liquid will then start rapidly boiling as it is being exposed to a temperature well above its boiling point and will then create a lot of gas, depending on its expansion ratio, and then the hazards of the gas are of immediate concern, especially if the incident occurs indoors.
It is always more desirable to have a puncture above the liquid level or a problem with the valve assembly; it is easier to deal with the gas phase than with the cold temperatures and the expansion ratios of a liquid leak. As pressure decreases, temperature decreases; the more rapidly this happens, the faster the cooling. Sometimes, a product will lose pressure so fast that the resulting temperature of the liquid will plummet below its boiling point and hold the product in the liquid state; this phenomenon is known as auto-refrigeration.
Cryogenic Liquids
Cryogenic liquids have boiling points at or below -130°F. They are gases that become liquid by cooling the compound below its boiling point and then storing it in a specialized cylinder or container that keeps it at that low temperature. These products are shipped as a tank within a tank. The tank holding the compound is surrounded by insulation in a vacuum-sealed space encased by a metal outer shell. Any failure that allows the liquid to warm will cause catastrophic tank failure and then the leaking product will expand according to its expansion ratio. There will be a significant danger of frostbite injuries and a massive accumulation of gas. Common examples of cryogenic liquids include liquefied natural gas, liquid oxygen, liquid helium, liquid argon, liquid hydrogen, liquid carbon dioxide (technically outside of the definition of a cryogenic liquid with a boiling point of -109°F but so cold that it is allowed into the category), and liquid nitrogen.
During normal operations, the piping coming from fixed tank at facilities is often wrapped in ice since the super-cooled liquid in the piping freezes the moisture in the air surrounding the piping (photo 1).
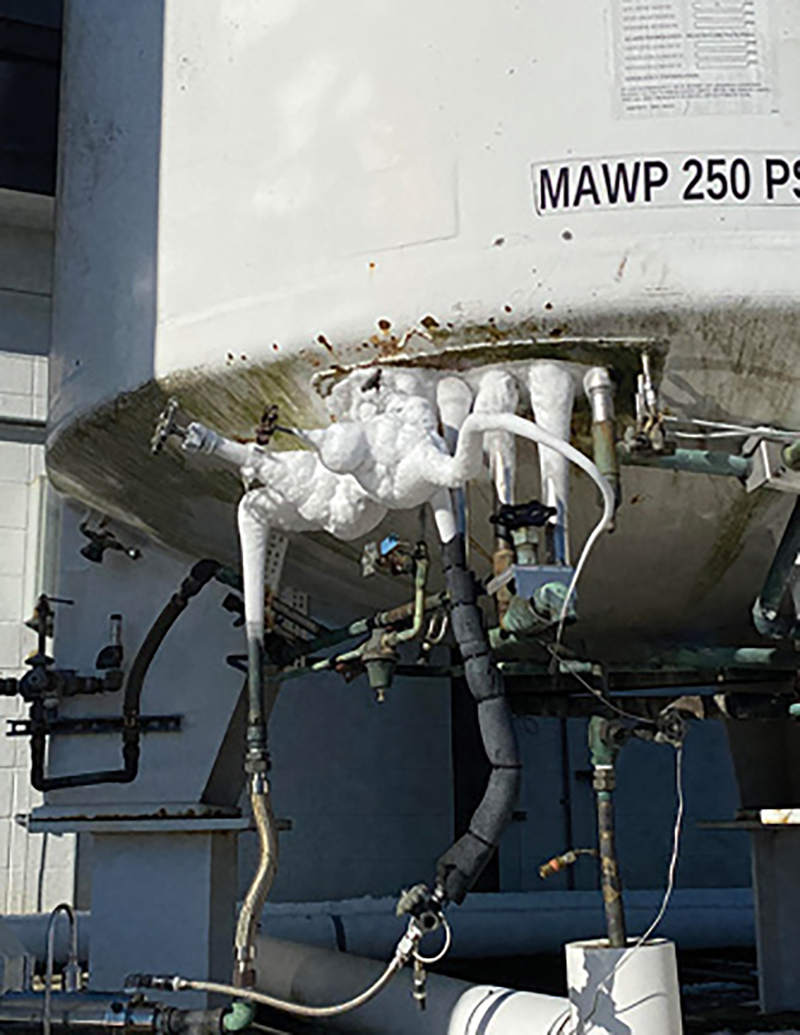
(1) Photos by author.
Aside from causing frostbite injuries, many cryogenic gases displace oxygen on release, making them simple asphyxiants. They can be flammable, oxidizers, and toxic and can cause metal embrittlement of surrounding structures. The inner tank holding cryogenic liquids is made of special steel alloys containing nickel, which allows the metal to maintain ductility at extremely cold temperatures. The outer metal shell is constructed of steel. Leaking cryogenic liquid will pool around the tank as it is rapidly boiling into a gas. When this super-cold liquid contacts metals that are not designed for extremely cold temperatures, it makes the metal brittle. This now brittle metal could shatter or snap, causing injuries if that metal (e.g., the bolts and supports for the cryogenic liquid tank itself) is supporting heavy objects or other tanks of hazmats (photo 2-3).
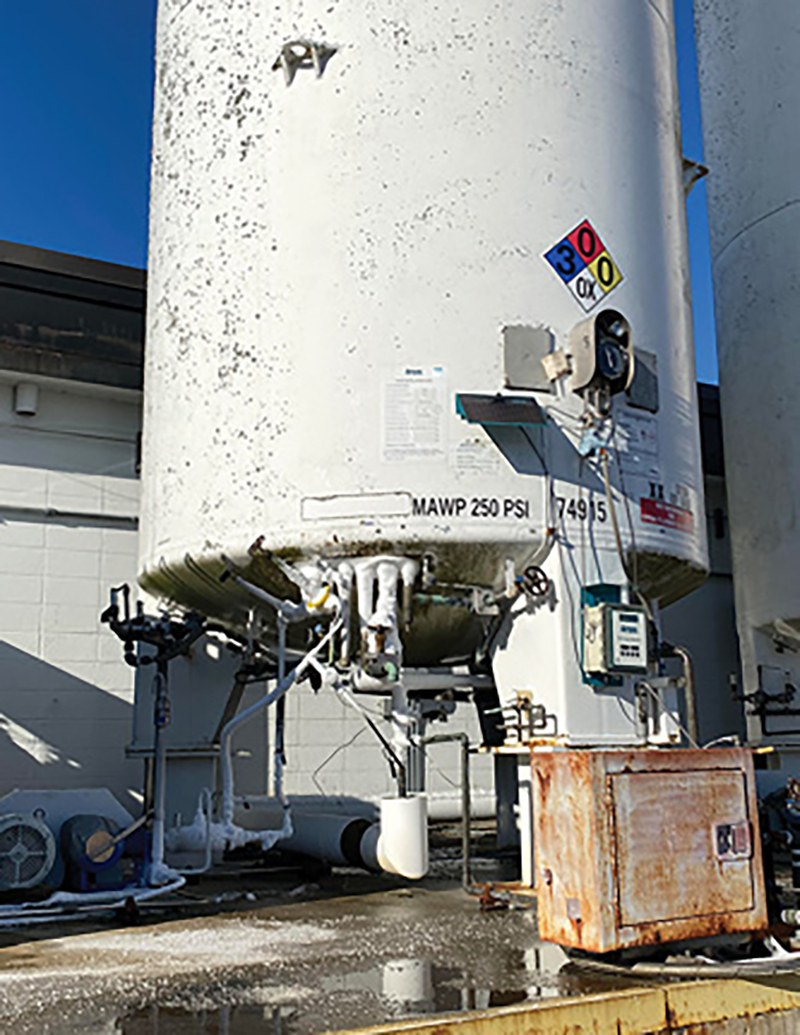
(2)
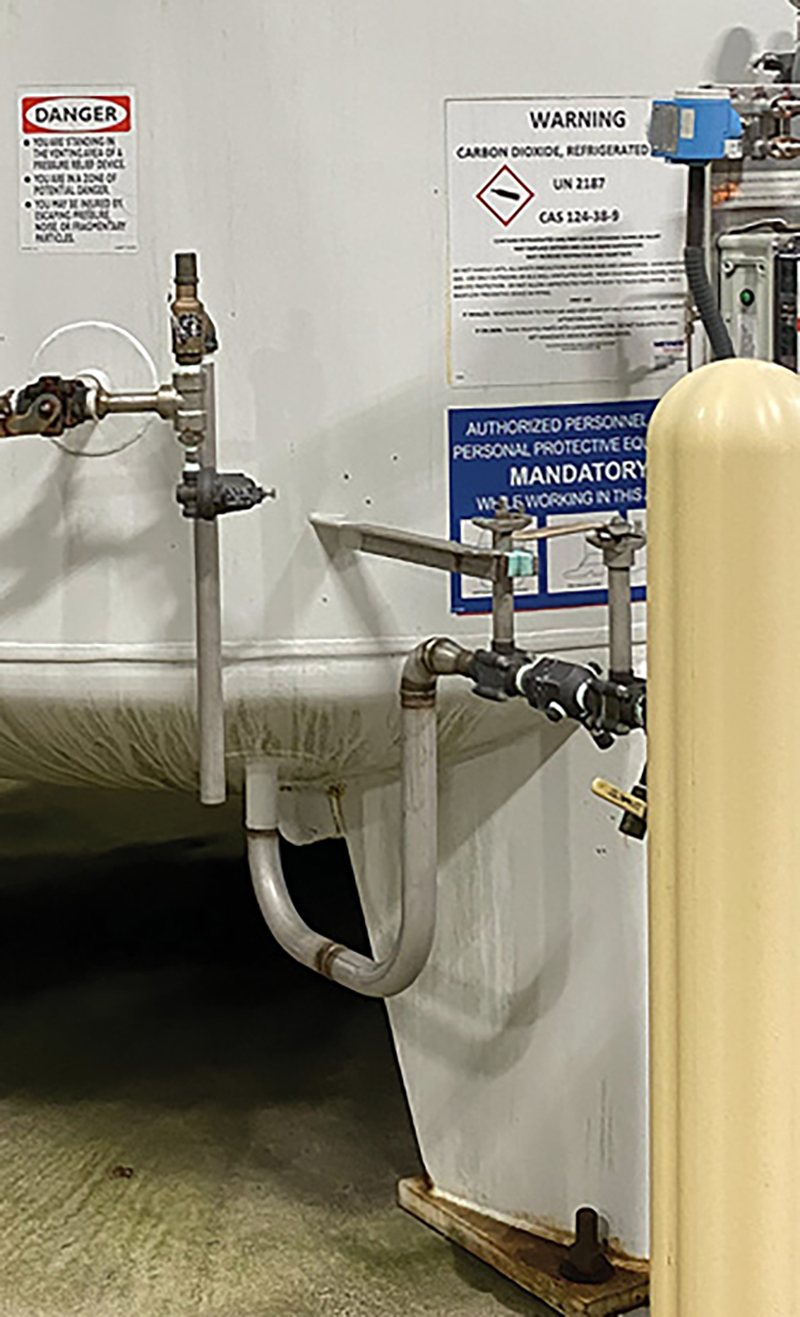
(3)
Liquid oxygen spills create oxygen-enriched atmospheres, increasing the flammability of exposed materials by decreasing the amount of energy needed to cause ignition. Oxygen enrichment can ignite flammable materials from ordinary combustibles to surfaces contaminated with hydrocarbons with much greater intensity than normal combustion. If liquid oxygen comes in contact with asphalt, the fuel and oxidizer mixture only requires a minute amount of activation energy, such as the friction of a footprint, to cause a detonation.
Lastly, liquid helium is so cold that air exposed to transfer hoses containing it can condense it to liquid nitrogen and liquid oxygen. This liquid oxygen condensate can contribute to oxygen-enriched atmospheres or ignite flammable materials that it comes in contact with.
When liquefied gases are released in the liquid phase, the rapid boiling of the liquid into gas will initially leave the gas cold until atmospheric conditions warm it. As a result, this super-cold gas, even if it would usually rise (if it has a vapor density of less than 1), may initially be found closer to the ground. The area of the release will be concentrated near the ground, and then the gas will rise farther away from the release as it warms. The release of liquefied gas can be deadly; understanding the physics of these gases allows responders to protect themselves from frostbite and asphyxiation.
On the surface, oxygen, carbon dioxide, helium, and argon may seem like they cannot hurt responders, but this is far from true when these gases are liquefied. Oxygen can permeate the layers of structural firefighting gear, making the garment flammable if the wearer responds to a house fire. Take liquid oxygen leaks seriously, and never treat them as simple to handle as the compressed oxygen tank found on the ambulance.
Chemical Properties
Although physical properties outline changes in states of matter but not the actual compound itself, chemical properties involve the changing of the original compound to something different. Chemical properties reflect how a material can harm. The most common chemical property firefighters encounter regarding gases is flammability. Flammable gases will make flammable mixtures with oxygen in air; in the right ratio with activation energy, they can explode or combust rapidly. Gases can also react with other chemicals to produce new compounds with their own dangerous properties. Use extreme caution with placards and labeling. At least, research the chemicals using the U.S. Department of Transportation (DOT) Emergency Response Guidebook (ERG) (2020 ed.) as a start (https://www.phmsa.dot.gov/training/hazmat/erg/emergency-response-guidebook-erg). A new edition will be published in 2024. Note that a material may be classified one way for shipping purposes but may be hazardous. For example, anhydrous ammonia is a gas designated with a UN 1005 green nonflammable gas placard but only for shipping purposes because of a U.S. DOT definition (photo 4). However, anhydrous ammonia is absolutely flammable, and the ERG clearly states this.
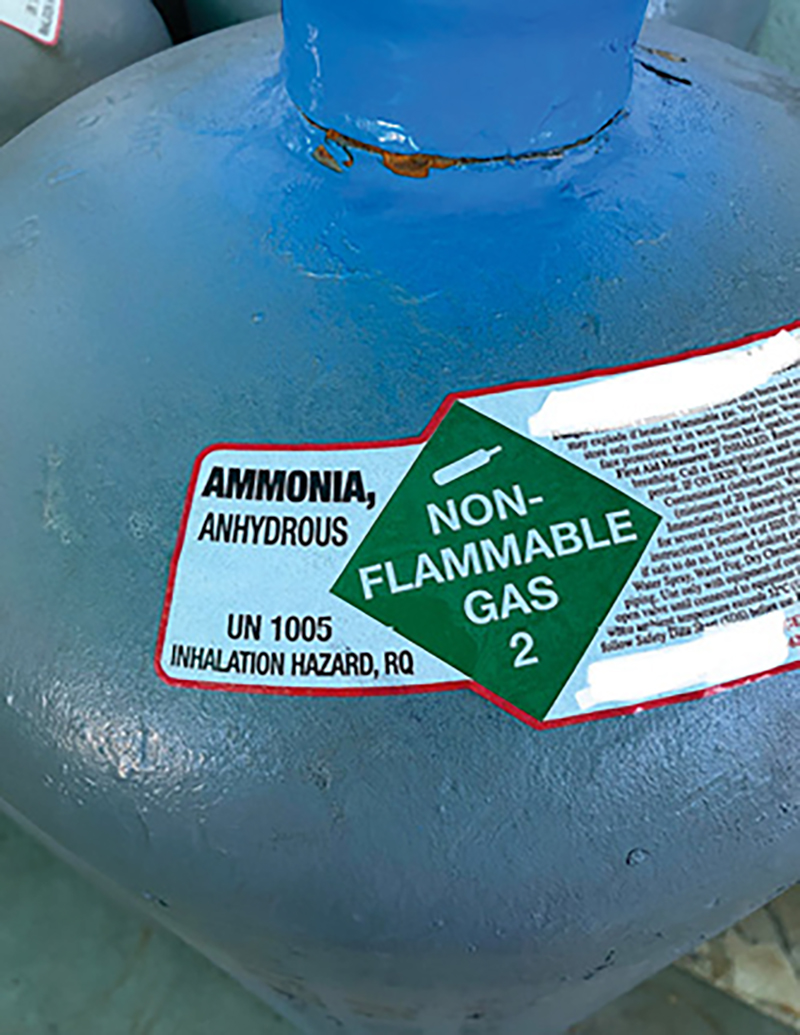
(4)
Flammable Range
When a flammable gas and oxygen combine in air, they create a flammable mixture, but each flammable gas will do so only in a specific range, the flammable range. The mixture must be between the lower explosive limit (LEL) and the upper explosive limit (UEL) to combust. If the concentration of gas is below the LEL, the mixture is too lean to burn; if it is above the UEL, the mixture is too rich to burn.
The most common gas first responders encounter is natural gas, comprised mostly of methane, and therefore its flammable range follows that of methane. The LEL is 5%, the UEL is 15%, so the flammable range is 5-15%. The closer the gas concentration is to the LEL and UEL, the less powerful the explosion; the closer to the midpoint of the flammable range, the more powerful the explosion will be if an ignition source is found. On ignition, the combustion process changes the original chemical into new compounds. Among the many reasons acetylene is so dangerous is that it has a flammable range of 2.5-100%, one of the widest ranges found in a product so commonly used.
Oxidizers
Many gases do not burn but can support combustion. This is a dangerous chemical property because when oxidizers are present, fires can burn with much more intensity. Also, the required activation energy to initiate combustion is significantly reduced when flammable materials are exposed to oxidizers. Chlorine gas is placarded as an oxidizer; despite being extremely toxic, it can support and increase combustion reactions. Oxygen is the oxidizer that is most prevalent in any community, especially where liquid oxygen is present. Handle liquid oxygen leaks with extreme caution. The dangers include frostbite, oxidized fuels igniting, and the saturation of structural fire gear with oxygen molecules. First responders to liquid oxygen leaks should stage upwind, control ignition sources if they can do this safely, use fans from a distance to create air currents to help disperse the oxygen into the atmosphere, isolate, deny entry, establish a command structure, and call for a hazmat team response.
Corrosives and Chemical Reactions
A corrosive gas can be classified as an acid or as an alkaline (caustic, a base). The only alkaline or caustic or basic gases are anhydrous ammonia and ammonia derivatives; all other corrosive gases are acidic. Corrosive gases are among the most dangerous compounds that hazmat responders will face and require chemical vapor protective clothing (Level A) to mitigate. Corrosive gases react with water to create corrosive solutions, which is how these gases harm humans. They turn any water from the body, whether in the eyes, the respiratory system, the armpits, the groin, or any perspiration on the skin, into a corrosive solution.
Chlorine is an oxidizer but also a corrosive. When it reacts with human moisture, it creates hydrochloric acid and hypochlorous acid. Ammonia reacts with water to create ammonium hydroxide. Hydrogen chloride gas reacts to create hydrochloric acid. All these corrosive solutions will significantly damage human tissue. Gases will react with the liquids and the solids they come in contact with or can react with other gases, sometimes violently. Ammonia can react with hydrogen cyanide gas to create intense heat that could cause fires as well as a polymerization reaction (a rapid chain reaction liberating heat and pressure). Any hazmat research should always include substances with which the compound could react. In some research materials, such as the National Institute for Occupational Safety and Health Pocket Guide to Chemical Hazards, this section is called “Incompatibilities and Reactivities.” It is listed on every chemical’s information sheet in the book. Known incompatible materials should always be kept apart from each other:
- Keep cyanides away from corrosives.
- Keep acids away from bases (alkalines, caustics).
- Keep flammables away from oxidizers.
Many substances are unstable; reactivity for them is a matter of temperature, pressure, or a failure in the systems that keep them safe. For example, always store acetylene cylinders upright. The gas is contained in its cylinder with acetone to help stabilize it, and the inside of the tank has a structure built into it to keep the gas from concentrating into one area. If allowed to do so, the compound can become extremely unstable, react with itself, and rupture the tank. Pressurizing acetylene to two atmospheres (29.4 psi) will cause it to explode.
Research chemical properties thoroughly at any hazmat incident since these properties of a gas cause the greatest harm to responders and the public.
BLEVE
Every fire service recruit has seen the short training video about a boiling-liquid, expanding-vapor explosion (BLEVE). Firefighters are taught to cool the tanks of hazmats that are exposed to direct flame impingement if it is safe to do so. Often, unmanned master streams must be employed while the area is evacuated. Firefighters are also taught to recognize signs of impending tank failure, such as the activation of pressure-relief devices, bulges, cracking, or noises such as pings and banging sounds. Depending on the type and size of container, pressure-relief devices can include pressure-relief valves (photo 5), frangible discs (photo 6), and fusible plugs (photo 7).
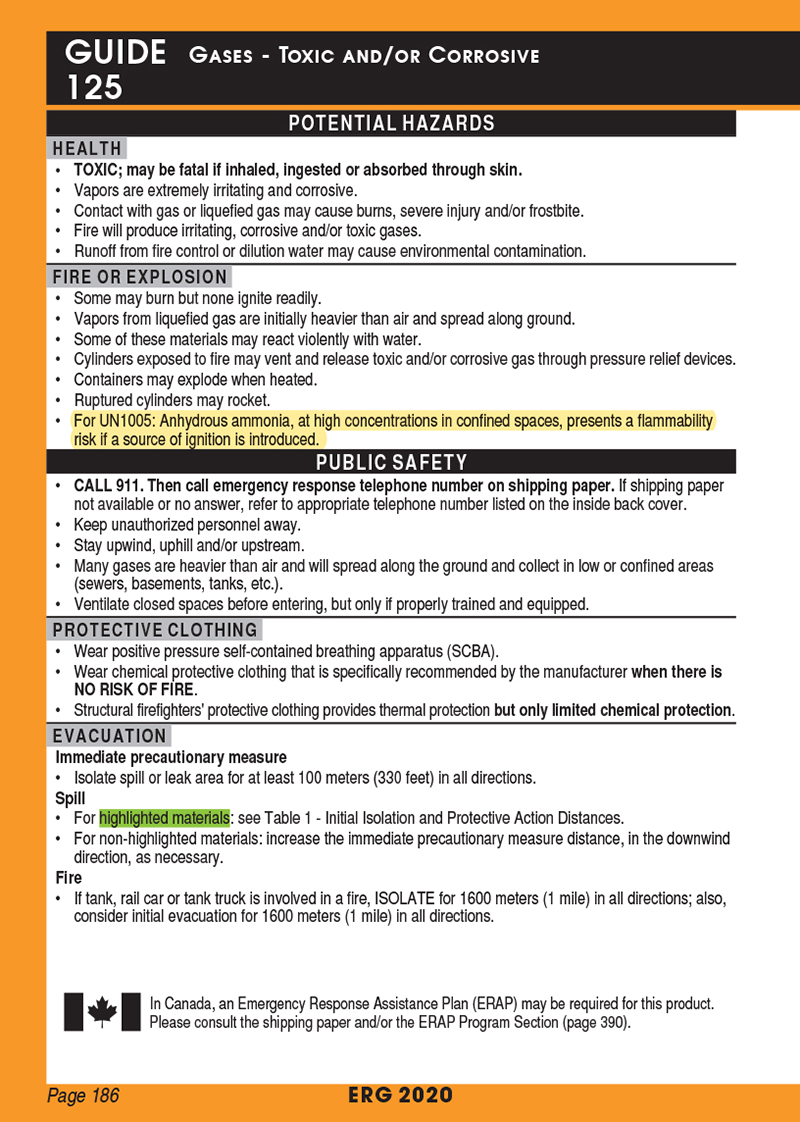
(5)
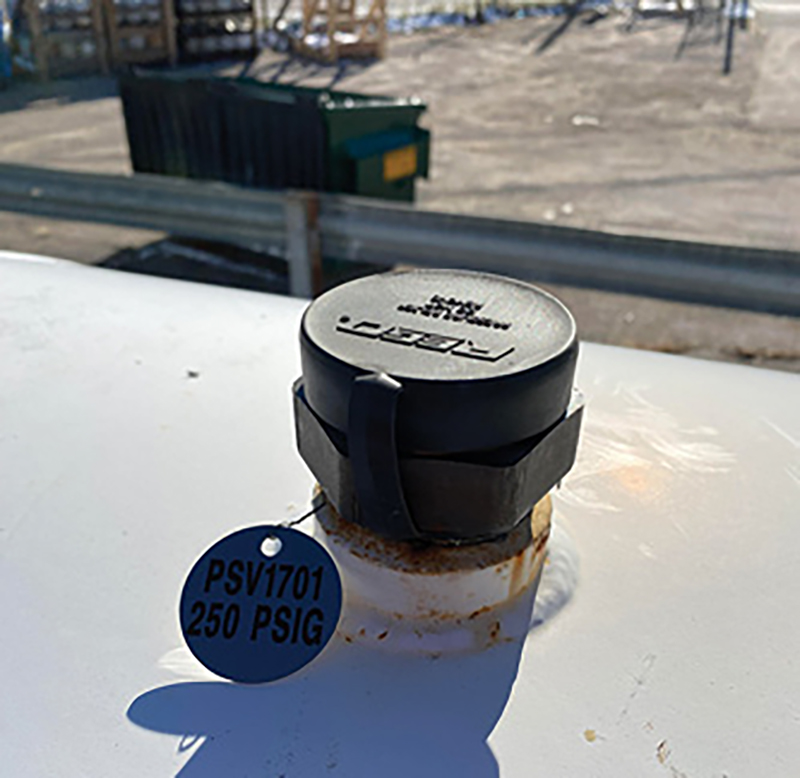
(6)
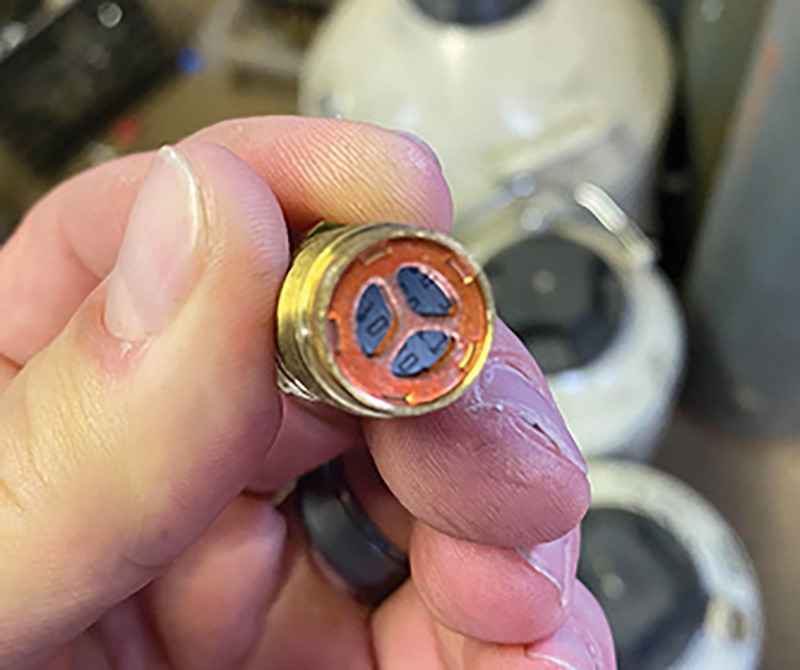
(7)
For liquefied gases, the liquid phase of the gas will act as a heat sink, but the vapor space above it must be rapidly cooled at the point of impingement since that space is rapidly increasing in pressure, which will lead to a catastrophic tank failure and ignition of its contents if it is flammable with explosive force. Even if the compound involved was not flammable, the explosive force of a BLEVE comes from the expansion ratio. Water has an expansion ratio of 1:1,700; if water is the boiling liquid inside of a container and the tank pressurized to the point of failure, rapid expansion of the liquid to a gas occurs with explosive force, propelling the tank or components of it with potentially deadly results. Aside from the classic understanding of a BLEVE, the knowledge about physical and chemical properties from above will reveal that there are other ways that a tank can fail, not just from flame impingement.
Chemical processes can involve the heating of materials in piping, columns, pressure vessels, and reactors. If there were a failure in the process system that a pressure-relief device could not control, the system could be overpressurized and subsequently fail in a BLEVE scenario. When filling cylinders, if the technician filling the tank with liquefied gas overfills past the 80% safety bumper, then the liquid in the tank can expand and overwhelm the design pressure of the tank, causing failure. The overfill prevention device (OPD) valves address this problem; these valves are identified by their triangular shape (photo 8). Using propane as an example again, the technician filling the tank uses weight to determine when to shut off the flow. If the technician is not paying attention, the OPD float would rise with the liquid and, at the 80% mark, would prohibit any more product from entering the cylinder (photo 9).
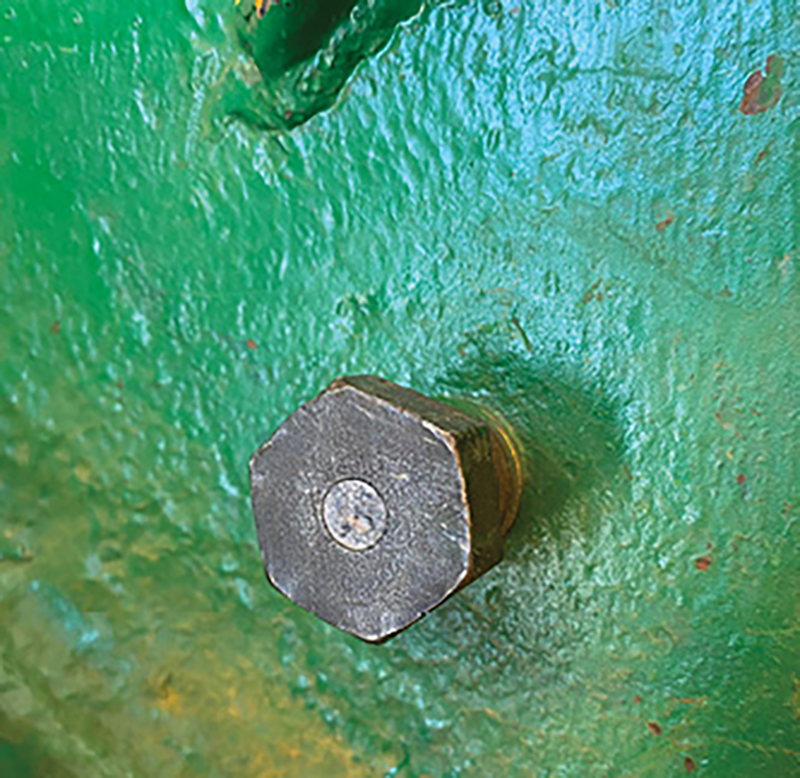
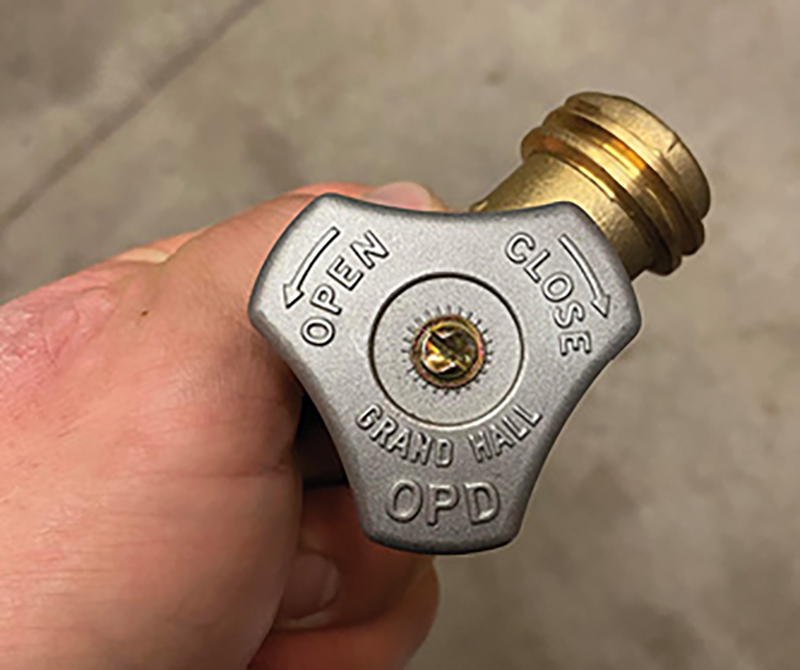
Cryogenic liquid containers can be subject to pressure increases if the outer shell or insulation space is compromised. Cryogenic liquids have boiling points at or below -130°F; any failure that allows the compound to be subject to ambient temperatures will cause the product to boil. This can potentially lead to an increase in the internal tank pressure and subsequent failure of the tank. It is not uncommon to see the pressure-relief devices on cryogenic cylinders, even those as large as tank cars, activate periodically. The venting gas is so cold that it appears white as it condenses the moisture in the air.
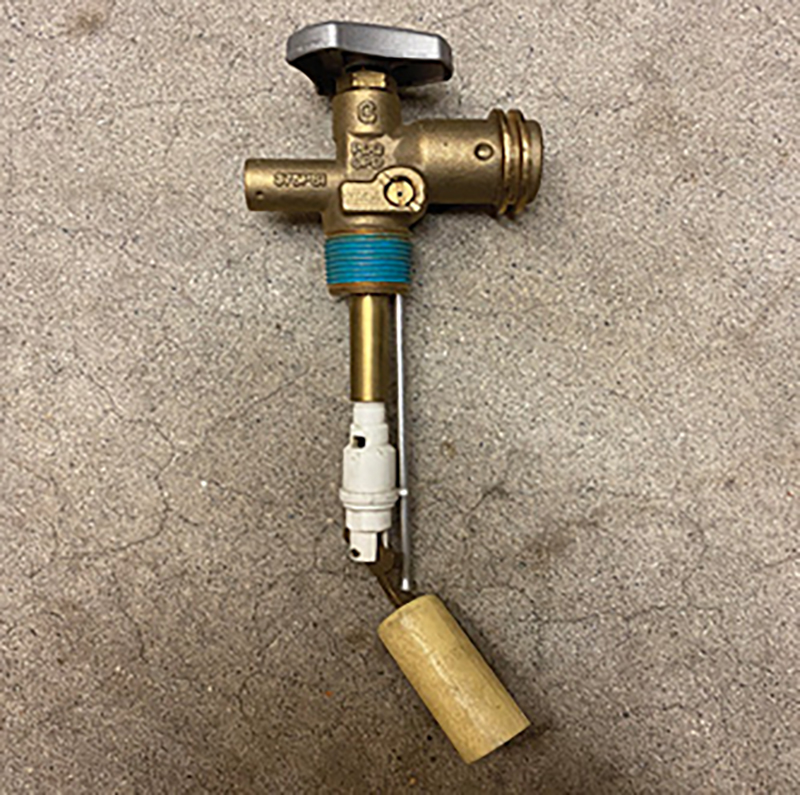
Finally, if a product is loaded into a tank that is not compatible with the container’s materials of construction, the resulting chemical reaction can cause tank failure. Likewise, if a container was loaded before it was properly cleansed of the product it previously held, the two compounds mixing could cause a reaction and subsequent tank failure.
Nothing in life exists without chemistry; even the human body is a nonstop machine of chemical reactions. The modern world would not exist without humans thoroughly understanding and using chemistry. Modern firefighters cannot keep themselves or the public safe without competence in how chemistry can hurt people. Firefighters encounter hazmats in some form on almost every incident; gases are not only prevalent but pose the greatest risk to health and safety. Understanding the chemical and physical properties of gases and how these properties will be encountered in the field, especially during emergencies, is imperative to keeping responders and the public safe. Companies should become familiar with the gases in their response district and, with the information contained in this article, can perform research on them and develop a preplan should an incident occur.
RICHARD C. BEAULIEU is a lieutenant on Rescue 1 for the Cranston (RI) Fire Department (CFD), where he has served since 2008. A 17-year veteran of the fire service, he has a bachelor’s degree in fire science from Providence College. A hazmat technician since 2006, Beaulieu is an NFPA 1041-certified instructor and coordinates the hazmat technician training for the CFD’s recruit training academy.