BY Nicholas Papa
The fire triangle identifies the mutual relationship among fuel, oxygen, and heat—working together to support combustion. Yet, the message of this most fundamental fire behavior lesson is often lost and not translated to the fireground. As a result, the actions taken or not taken can result in conditions worsening initially on the arrival of the fire department, particularly regarding ventilation. “Proper ventilation is one of the most essential, yet one of the most poorly practiced firefighting tactics.”1
To select and properly execute the most appropriate interventions, we must understand how the three elements of the fire triangle are connected and how they impact the fire’s behavior; its environment; and, most importantly, people within it—particularly the unprotected victims. “There is a distinct difference between knowledge and understanding; possessing a collection of the two in balance is how you achieve wisdom.”2 With a solid foundation of the scientific principles behind the tactics we perform, we can then constructively validate our experiences and refine our mental performance, the key to developing wisdom. “The why is the missing component in the fire service …. When we understand the why, we can solve the problems that are going to occur on the fireground.”3
Fuel and Heat
The energy that a fuel produces as it is consumed by fire is known as the heat of combustion. Every fuel has a defined heat capacity, which is determined by its chemical makeup, referred to as the potential energy of fuel.4 It is widely known that synthetic fuels (plastics/polymers) have a much greater energy (heat) potential than their cellulosic counterparts (wood and natural fibers)—in excess of two times the amount measured in watts (power) and Joules (power/time). Although this is certainly important, it is the increased speed at which the synthetics liberate their heat that is of far more concern. The greater the heat release rate (HRR) of the fuel load, the faster the temperatures will climb within the compartment.
The HRR of a given fuel is dictated by four characteristics: surface-to-mass ratio, orientation, arrangement, and geometry.5 As the HRR increases, the following detriments are experienced: accelerated combustion (decreasing the time to flashover), increased temperatures, production of fire gases, and lowering of the oxygen concentration. This factor then becomes the catalyst for deteriorating conditions within the compartment, promoting fire development and reducing tenability of the survivable space, making it clear why HRR is referred to as the “single most important variable in fire hazard.” (5)
Oxygen and Heat
For fuels to achieve peak HRR and liberate their full energy potential, a sufficient oxygen supply must be available to the fire. The recent fire dynamics research has brought attention to this fact, but the concept is not at all new. In fact, the fire service documented this correlation 87 years prior to its being published by the scientific community. In 1830, James Braidwood, Master of Fire Engines in Edinburgh, Scotland, wrote: “… the door should be kept shut while the water is being brought, and the air excluded as much as possible, as the fire burns exactly in proportion to the quantity of air which it receives.”6 Nearly a century later, W.M. Thornton determined that for each unit of oxygen consumed for combustion, there is a fairly constant heat output among common hydrocarbon fuels and their derivatives, commonly referred to as “Thornton’s Rule.”7,8
Figure 1. The Fire Triangle Represents the Three Elements of the Combustion Process
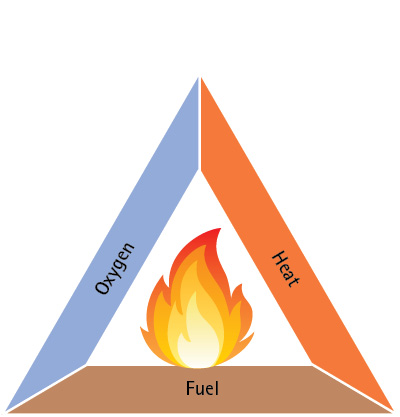
Oxygen and Combustion
The air that is readily available for combustion, known as the air/fuel interface, is determined by the previously identified physical characteristics of the fuel load. The supply of air is regulated by the dimensions of the compartment (volume); the available openings (inlets and outlets) and their orientation; and the subsequent flow types, known as the ventilation profile.9 As the fire grows, so does its demand for oxygen. When the concentration of oxygen within the space begins to diminish (falls below 15 percent), so does the fuel load’s ability to release its potential energy and eventually to sustain combustion altogether.
Based on the average HRR experienced in a typical compartment fire, the oxygen supply in an enclosed 8-foot × 12-foot × 8-foot space (a common bedroom size) would be consumed in just over two minutes.10-12 (4) Although the combustion process is not linear and the volume of air is not fixed (an additional supply may be provided from external openings and adjoining compartments), this example demonstrates how rapidly the present environment can impact the oxygen supply and, consequently, the fire’s behavior.
In the absence of the required air flow, the fire will remain underdeveloped in a vent-controlled state, which is predominantly the case given the fuel-rich contents and energy efficiency of today’s fire environment—increasing the oxygen demands while limiting the air exchange. “Many, if not most, fires that have progressed beyond the incipient stage (when the fire department arrives) are ventilation controlled …. [the fire’s power] is limited by the existing openings.”13
Figure 2. The Growth Curve of a Ventilation-Controlled Fire
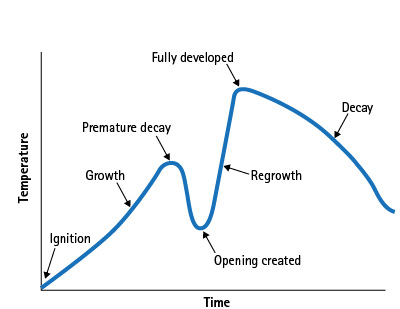
If the fire lacks an adequate oxygen supply, the fire will enter a premature decay stage—suppressing combustion and eventually resulting in self-extinguishment. With the fuel load and the heat still present, however, this condition can pose a threat if the necessary precautions are not taken, setting the stage for a potential backdraft or ventilation-induced flashover.14 If an (uncontrolled) opening is created (without the prompt application of water or management of the air flow), the sudden intake of fresh air can cause the fire to reenergize rapidly in a second growth stage and begin to deteriorate conditions in just seconds, as demonstrated in the Underwriters Laboratories (UL)/National Institute of Standards and Technology test fires. (13) As Chief John Norman so poignantly stated, “Whoever controls the supply of oxygen wins the battle.”15
The Movement of Air and Fire
The speed and intensity with which the fire reacts to ventilation vary and are contingent on several factors: the conditions within the compartment (temperature and fuel load), the characteristics of the openings (location and size), the weather (wind velocity), and the resultant air flow/exchange (ventilation profile). The higher the interior temperatures, the greater the fuel load; the closer in proximity to the fire, the larger the openings; and the more efficient the air flow (unidirectional over bidirectional) determined by the orientation of the openings, the faster the fire will respond to the ventilation, amplified by a prevailing wind condition.16-17
Figure 3. The Exothermic Reaction Produced by the Combustion Process

Figure 4. The Intermediate Neutral Plane at the Opening Identifies a Bidirectional Flow Within
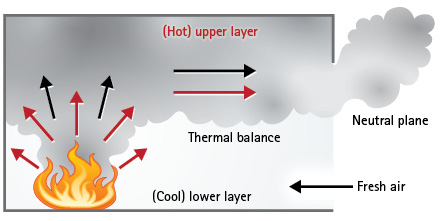
Being an exothermic reaction, fire produces energy. On being heated, the gases expand and generate pressure within the compartment. (15) The movement of air will always proceed from areas of high pressure to those of lower pressure, taking the path of least resistance. UL refers to the route by which the air travels as the flow path, defined as, “the volume, composed of area(s) within a structure, between an inlet and an outlet that allows the movement of heat and smoke from the higher-pressure fire area towards the lower pressure areas via doorways, stairways, or windows.”18 In the mid-1800s, Braidwood first brought this fact to light, originally identifying the pattern of air migration as the “draught”’ (draft). As the fire develops, its by-products (heat, smoke, and gases) will rise and expand outward, moving away from the area of high pressure, and will begin to bank down as they reach the walls of the compartment. (16, 17)
The Effects of Ventilation
When an opening is created on a plane with or above the area of involvement, the fire and its by-products will be drawn to that area of low pressure. “Ventilation creates a draft effect and may pull fire either vertically or horizontally.”19 If the opening or a portion of it is above the neutral plane (the horizontal dividing line at an opening where fresh/cool air flows in from below and the by-products of combustion discharge above), it will begin exhausting the by-products of combustion, which will create an inverse (air flow) reaction. As the by-products of combustion are discharged, any opening (or portion of it) below the neutral plane will proportionately allow greater amounts of fresh air into the compartment.
If an opening encompasses an area above and below the neutral plane, it will function as both an inlet and an outlet, resulting in a bidirectional flow. This is also not a recent discovery: Chief Lloyd Layman wrote in 1955: “… open or burned out windows can serve as both exhaust and air-intake openings. Heated smoke is exhausted from the upper section while cool air from the outside atmosphere enters through the lower section. This action also occurs through an open doorway.”20 When an opening resides entirely above the neutral plane, it will serve as an outlet, producing a unidirectional flow. The higher up an opening is, the more efficient the air flow/exchange will be. Conversely, an opening situated fully below the neutral plane will function as in inlet.
Figure 5. An Opening on the Plane with and Above the Fire Creates a Unidirectional Flow, Allowing for Pure Exhaust at the Outlet

Ventilation Limitations
Every opening has a finite exhaust capacity and, therefore, a limited ability to confine the fire and its by-products. As the fire develops, the energy it produces can eventually exceed the exhaust capabilities of the opening created. Once the opening has been consumed, the fire will start to spread laterally, seeking other low-pressure avenues, potentially at an overwhelming velocity. “Once ventilation is started, the time for effective extinguishment is limited.”21
Given the fuel load and configuration of a typical dwelling compartment, there are not enough existing openings (approximately 70 square feet for an average living room) to realistically contend with the output of today’s vent-controlled fires without being coordinated, making it “nearly impossible” to transition the fire to a fuel-controlled state exclusively through ventilation.22 Nearly 10 years earlier, Hossein Davoodi had drawn the same conclusion after determining that for a compartment to become fuel-controlled, at least 25 percent of the wall area would have to be opened up, totaling nearly 100 square feet of horizontal ventilation (based on the dimensions previously used), rendering such an approach impractical.23 Similarly, the standard (4-foot × 4-foot) vertical ventilation opening is capable of exhausting only the heat equivalent of a sofa fire, a fraction of the total fuel load with an average residential living room. (16-17) For this very reason, it is of the utmost importance that ventilation be synchronized with door control and fire attack, managing the air flow and reducing the heat.
Ventilation Timing
Preemptive ventilation may enhance tenability momentarily by releasing some of the pent-up by-products of combustion and drawing fresh/cool air into the compartment at the ground level, potentially promoting a brief “lift” of the neutral plane (actually smoke tunneling caused by the inrush of air toward the underventilated fire). If water is not promptly applied to the fire or if the flow of air is not controlled until that time, however, the benefits, if any, can be short-lived. The reaction experienced will be dependent on the openings created and the conditions present.
Openings created remote from the fire (such as bulkheads/skylights, windows in adjacent/interconnected spaces, and even entry doors) can draw the fire to that point of lower pressure if the area is not isolated prior to the onset of extinguishment. This is the reason controlling the door is of the utmost importance when performing vent-enter-search (VES). “… venting for life brings the inherent risk of pulling the fire in your direction. Although essential, it must be performed for a specific reason.”24 Regarding vertical (bulkhead) ventilation, “… if the line is moving in or the truck has door control on the fire floor, vent (the bulkhead). If they do not, then coordination is required with the officer at the tip of the spear … differentiate between known versus suspected life hazard. If you see/hear something or a credible witness on the fireground says there is someone in there, do your job to the best of your ability to save them.”25 In these instances, the (bulkhead) door can still be forced to gain access and promptly conduct a search of the (stairwell) landing, if the door is immediately controlled after entry. The door should remain shut until authorization is received or until certain benchmarks have been achieved.
The point of diminishing returns can onset rapidly and abruptly. You may decide not to vent a burning building when the hose team is not ready to advance, and premature venting of a window or a door may spread fire or increase its size. (14) As the window of effectiveness for ventilation continues to narrow as the fireground evolves, so does our margin for error. Because there is no reliable way of accurately predicting the duration of that lag time once ventilation is executed, it is of the utmost importance that ventilation be coordinated—it requires fireground discipline.
Coordination and Tempo
Evaluating conditions and the progress of interior crews is paramount to properly selecting the appropriate ventilation interventions. Successful execution, however, is contingent on accurately reading the situation and its rate of change and establishing the correct operational tempo, defined as “speed relative to a problem set.”26 To aid in this process, there are four steps that should be taken in the following order: (1) read the ventilation profile; (2) anticipate its impact; (3) determine the operational needs; and (4) execute [and reevaluate]. (9) There is a direct parallel between this strategy, developed by Paul Grimwood and Colonel John Boyd’s OODA Loop: observe, orient, decide, act.27
The purpose of our tactics is to positively influence the environment/fireground to “make it behave” and allow us to gain the upper hand. Fireground tempo is “a series of disciplined, appropriate and timely tactical actions that promote mission accomplishment and enhance survivability … performed at a rate quicker than the environment’s (fire’s) rate of change.” (26) As we operate, most notably in the performance of ventilation operations, we must ensure that we outpace the fire, not ourselves. We must understand the impact and limitations of our ventilation tactics and acknowledge that we cannot practically out-vent today’s vent-controlled fires; it must be accompanied by effective water application. Determining the proper location, timing, and amount of ventilation is fundamental to accomplishing this task.
Figure 6. The Parallel Between Colonel Boyd’s OODA Loop and Grimwood’s Tactical Ventilation Strategy
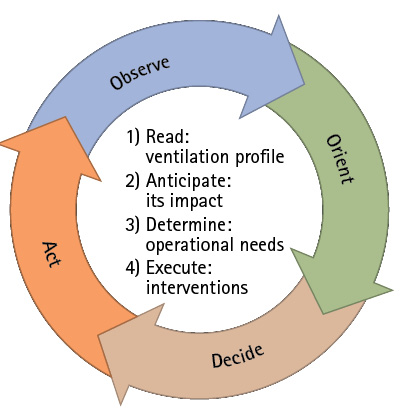
Ventilation is not a standalone tactic; it is a supportive function and a coordinated effort intended to augment firefighting operations and enhance victim survivability. “Although we cannot control all aspects of ventilation, proper ventilation techniques can make for safer operations as well as increase survival time for occupants and victims.” (24) To improve our chances of success, we must ensure that ventilation remains strictly task-orientated (having a clear objective); is executed in cadence with the progress of fire attack and search crews; and remains aligned with the mission of preserving life and property.
References
1. Fornell, David. (1991). Fire Stream Management Handbook. Pennwell Publishing: Saddle Brook, NJ.
2. LeGear, Dennis. (2017). Social media post on May 28, 2017. Retrieved from https://www.facebook.com
/photo.php?fbid=435139000194824&set=
pcb.435139030194821&type=3&theater.
3. Fields, Aaron. (2017). Fire Engineering BlogTalk Radio: The PJ Norwood Show. Moving Forward Presentation at Andy Fredericks’ Training Days.
4. Hartin, Ed. (2009). Thornton’s Rule. Retrieved from http://cfbt-us.com/wordpress/?tag=thorntons-rule.
5. Babrauskas, V. and Peacock, R.D. (1992). “Heat Release Rate: The Single Most Important Variable in Fire Hazard.” Fire Safety Journal.
6. Braidwood, James. (1830). On the Construction of Fire-Engines & Apparatus & the Method of Proceedings in Cases of Fire. Bell & Bradfute: London, England.
7. Thornton, W.M. (1917). The Relation of Oxygen to the Combustion of Organic Compounds. Philosophical Magazine & Journal of Science.
8. Crapo, W.F. (2011). Thornton’s Rule: Anticipating the Heat-Release Rate. Retrieved from http://www.
fireengineering.com/articles/print/volume-164/issue-5/features/thorntons-rule-anticipating-the-heat-release-rate.html.
9. Grimwood, Paul. (2017) Euro Firefighter 2. West Yorkshire, UK: D&M Heritage Press.
10. Svensson, Stefan. (2005). Fire Ventilation. Swedish Rescue Services Agency.
11. Madrzykowski, Dan. (2017). Fire Dynamics for Structural Firefighting Presentation. Retrieved from http://www.facebook.com/story.php?story_fbid=1929567690643700&id=1487961544804319&_rdr.
1 2. Vestal, Jason and Bridge, Eric. (2010/2011). “A Quantitative Approach to Selecting Nozzle Flow Rate and Stream,” Parts 1 and 2. Fire Engineering.
13. Hartin, Ed. (2011). Influence of Ventilation in Residential Structures: Tactical Implications Part 1. Retrieved from http://cfbt-us.com/wordpress/?p=1516. Tactics in Single Family Homes.
14. Dunn, Vincent. (2007). Strategy of Firefighting. Pennwell Publishing: Tulsa, OK.
15. Norman, John. (2012). The Fire Officer’s Handbook of Tactics. Pennwell Publishing: Tulsa, OK.
16. Kerber, Steve. (2010). The Impact of Ventilation on Fire Behavior in Legacy and Contemporary Residential Construction. Retrieved from http://www.ul.com/global/documents/ offerings/industries/buildingmaterialfireservice/ventilation/DHS%202008%20Grant%20Report%20Final.pdf.
17. Kerber, Steve. (2013). Study of the Effectiveness of Fire Service Vertical Ventilation and Suppression Tactics in Single-Family Homes. Retrieved from http://ulfirefightersafety .com/wp-content/uploads/ 2013/06/UL-FSRI-2010-DHS-Report_Comp.pdf.
18. Braidwood, James. (1866). Fire Prevention and Extinction. Bell & Bradfute: London, England.
19. Fried, Emanuel. (1972). Fireground Strategies. Pennwell Publishing: Saddle Brook, NJ.
20. Layman, Lloyd. (1955). Attack and Extinguishing Interior Fires. NFPA: Boston, MA.
21. McAniff, Edward. (1974). Strategic Concepts of Fire Fighting. Pennwell Publishing: Saddle Brook, NJ.
22. Zevotek, Robin. (2017). Accessing Ventilation-Limited Fires. Retrieved from https://modernfirebehavior.com/research-corner gaining-access-to-ventilation-limited-fires-by-robin-zevotek/.
23. Davoodi, Hossein. (2008). Confinement of Fire in Buildings. NFPA: Quincy, MA.
24. LeFemina, Fred. (2006). “Ventilation Basics.” Retrieved from https://www.fire rescue1.com/fire-products/ventilation/articles/18506-Ventilation-Basics/.
25. Ceriello, John. (2017). Social media post on September 22, 2017. Retrieved from https://www.facebook.com/photo.php?fbid=1876090579308249&set=
a.1553656024885041.10737
41828.100007219744897&type=3&theater
26. Brezler, Jason. (2017). Fireground Tempo lecture, April 22, 2017.
27. “The Observe, Orient, Decide and Act Model of Decision-Making.” (2012) http://www.firefighternation.com/articles/2012/01/the-observe-orient-decide-and-act-model-of-decision-making.html.
NICHOLAS PAPA is a lieutenant with the New Britain (CT) Fire Department and is assigned to Engine Co. 1. He previously was a private in Ladder Co. 2. He is a second-generation firefighter with more than 13 years of experience, including career and volunteer. He has a B.S. degree in public safety administration and an M.P.A. degree in emergency management. He is a contributor to Fire Engineering.