BY STEPHEN RHINE
Air-supported structures originated in the 1940s as a way to keep snow and ice accumulations off radars in Alaska and Canada. The snow and ice would interfere with signal delivery, as would a traditional brick-and-mortar building. The first “radome” (radar dome) constructed in 1946 was the first air-supported structure. Resembling a large balloon, the round covering was made of a neoprene-coated fiberglass fabric.
The first large-scale nongovernmental air-supported structure housed the United States pavilion at the 1970 World’s Fair in Osaka, Japan. The span of the dome was 262 feet by 460 feet, and the dome was less than 23 feet tall, meeting Japanese building codes at the time for withstanding typhoon winds.
:
Structural Design
Today’s air-supported structures are made from either a nylon fabric (typical in smaller applications such as tennis courts and ice rinks) or a polyvinyl chloride-coated woven polyester (used for larger applications such as soccer fields). Both membranes must comply with National Fire Protection Association (NFPA) 701, Standard Methods of Fire Tests for Flame Propagation of Textiles and Films, requirements regarding fireproofing. Regardless, the material used in these structures is very lightweight, usually measuring anywhere from 12 to 20 ounces per square yard. The seams of the material are heat melted in the factory at 300°F, so the seams may not maintain their strength with a significant heat source inside.
Air-supported structures employ two techniques. The first uses a heavier weight material for the membrane, which is bolted to the ground and inflated like a balloon, and gives the material its bubble shape (photos 1, 2). A more common technique uses a lighter weight material that uses a rib cast attached to a concrete footing over it. When the membrane is inflated, it takes the shape of the rib cast (photo 3). In both techniques, air pressure supports the structure. These structures are usually translucent to allow sunlight to filter through during the day and will have lights either affixed to the interior sides or hanging freely from the membrane.
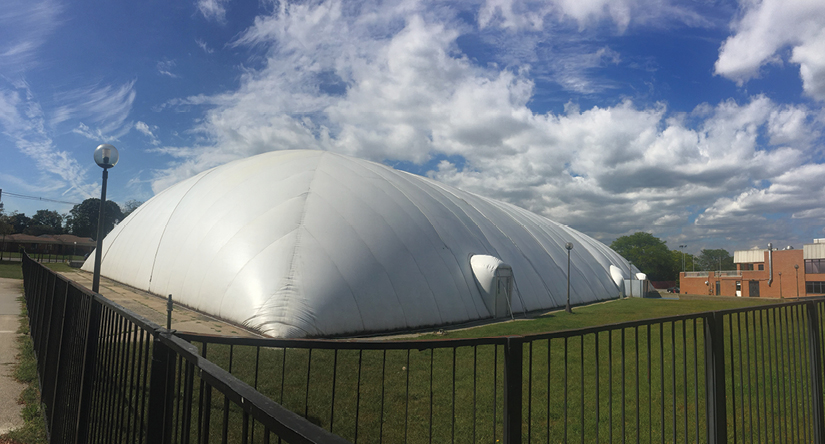
Photos by author.
Both designs require a constant airflow to keep the structure inflated; the air is provided by large heating, ventilation, and air-conditioning (HVAC) units that power high-output fans. These fans pump air into the structure using aboveground temporary ductwork or more permanent piping that usually runs under the structure to key locations within it. These facilities will also have a secondary diesel generator in case the power fails or a secondary inflation unit in case of the failure of the primary HVAC.
Air as a Support Mechanism
Air is used to support these structures because very low pressure will suffice to maintain its strength. Air molecules are composed of 21 percent oxygen and 78 percent nitrogen; traces of other gases make up the rest. As air molecules fly around, they bounce into each other in the same manner as two magnets with similar polarity will push apart. Like these magnets, the closer you put these air molecules to each other, the more force they will exert trying to separate. The air pressure in your car tires is a great example of these forces working to create strength. The more air that is pumped into a car tire, the more molecules there are pushing away from each other, thus supporting the sidewalls of the tire. The internal pressure of these structures is just slightly higher than the atmospheric pressure, which is what gives them their strength. The typical air pressure inside a car tire is 35 pounds per square inch (psi), while the pressure within an air-supported structure is only required to be .035 psi higher than atmospheric pressure.
Design Features
All structures have similar design features. Entrances will have either revolving doors or two doors with an airlock arrangement. Using these doors allows the structure to keep the proper internal air pressure when occupants are entering and exiting. This minor difference in air pressure is realized when your ears slightly pop while traveling through the air lock. Emergency exit doors are also integrated into their design. In an emergency within the structure, occupants may leave through a push bar-type exit door, allowing more occupants to leave at any one time. When these emergency doors open, the high-pressure fans will thrust more air into the structure for a predetermined time, depending on the occupancy limits for that facility. Without these fans running and the doors opened for occupants to escape, the design could fail completely within minutes, trapping occupants underneath the deflated fabric. Some structures may also have large garage doors to allow small machinery, such as scissor lifts and forklifts, to enter.
These complexes are designed to combat the elements. Since the top of the structure cannot support a drop of more than seven feet, the engineer on duty will raise the internal temperature and the air pressure the fans supply in an impending snowstorm. Air-supported structures are also designed to withstand the weight of eight pounds of snow per square foot.
If snow is accumulating on the structure’s roof, the engineer may throw ropes over the top to break up the snow and make it slide down the structure along a predetermined route. If too much snow accumulates on the structure’s top, an uncontrolled snow slide may occur. Such slides have been known to slice into the seam connecting the door to the membrane, causing it to tear open and expel the air, causing the structure to fail within minutes.
Also, snow is removed from around the structure to prevent large snowdrifts, which would press on the membrane and cause a puncture. Some larger structures have two layers of material between which hot air is pumped to warm the external layer enough to melt the snow and ice. Wind and snow are among the factors that have caused air-supported structures to collapse. During a snowfall, the structure’s internal temperature and air pressure are increased.
The International Building Code, Chapter 31, Special Construction,1 requires these air-supported structures to withstand a wind load of 90 miles per hour (mph) for a three-second gust. When the positive pressure from wind exceeds the internal pressure of the structure, the structure will begin to dimple on the windward side. This dimple may come in close contact with items within the structure, such as hanging lights or boards surrounding an ice rink, causing a puncture. When there is a strong wind weather advisory, the onsite engineer will increase the structure’s internal pressure.
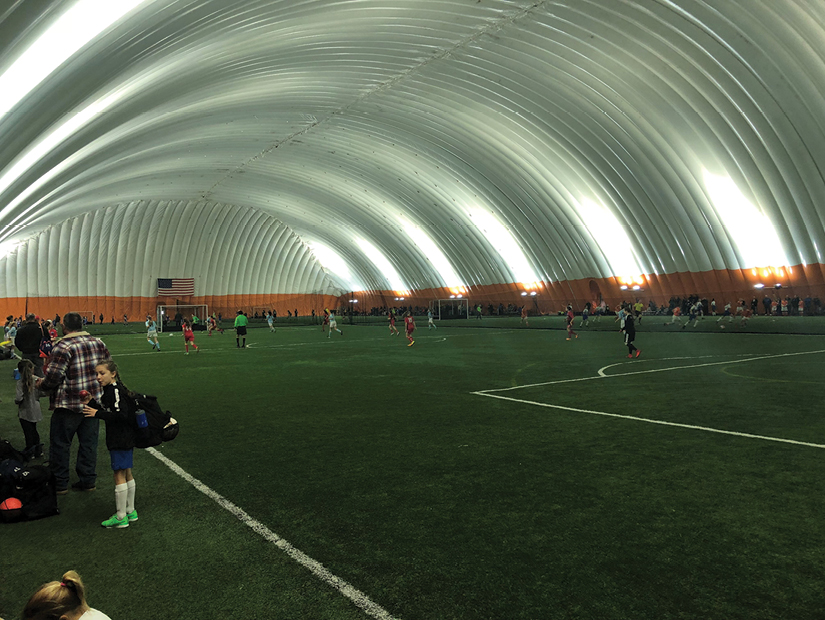
Air-Supported Studies
A University of Maryland study2 demonstrated that a fire in an air-supported structure would eventually create a small tear directly over the heat source. In the study, this small tear expanded quickly, diminishing the internal pressure and causing the structure’s complete failure. In the study, the tear occurred 10 minutes after igniting the controlled fire, which may allow a significant number of occupants to escape the complex before failure. In this study, however, the secondary fans installed were not large enough to maintain the structure’s configuration, and it deflated.
A Fire Research Station study3 showed that when a fire tears a hole directly above the source, all the smoke and heat would travel up and through that opening. However, when the doors open to allow occupants to escape, the larger door openings will cause the heat and smoke to exit in that direction, hindering the evacuation.
Response Considerations
When arriving on the scene of a deflated structure, determine if this is an emergency (it might be just a power loss) or a catastrophic failure of the HVAC or membrane. If a small fire occurs within the structure, chocking open doors may be detrimental to the operation. Instead, leave the door slightly ajar; even possibly allow the door to close on a charged hoseline to assist in maintaining the facility’s internal air pressure. Another option will be to contact the on-site building engineer and ask for an increase in the pressure in the fans.
Larger fires are best fought from the outside when deflation of the structure has caused the fabric to lie on the ground. However, items within this now-deflated structure may prevent the material from lying directly on the ground and may result in more complicated issues.
Air-supported material is classified as “not easily ignitable,”4 so there should be no difficulty in walking over the deflated structure to reach the seat of the fire. The material is easily cut with a utility knife or seat belt cutter when fully inflated. However, when deflated, the firefighter must exert more strength since the structure has lost its form.
While walking on top of a deflated structure, be aware that objects or victims may be beneath the fabric. Keep in mind that the large lights and electrical wires that were once hanging overhead have now crashed with great force. Also, when using saws to cut cables or rib cast away from the deflated structure for better access, keep in mind that trapped occupants and live electrical wires may be just beneath what you are cutting.

Causes of Collapse
Even with strict local building code enforcement, weather will likely play a major role in the collapse of these structures. Natural occurrences caused the collapse of air-supported structures at Arizona State University (2008, strong winds), the Philadelphia (PA) Port Authority (2002, snowfall), the Dallas Cowboys practice facility (2009, wind), and the New York Giants training facility (2007, strong winds).5 In the New York Giants Newark, New Jersey, practice facility, strong winter winds moved through the area and caused a set of revolving doors to detach from their foundation, creating a gap for air to rapidly escape. The Dallas Cowboys facility saw winds of 70 mph rip through the Valley Ranch area, destroying the facility. Steel supports that held the form of the structure came crashing down. As a result, 12 people were hospitalized, including a scouting assistant who became permanently paralyzed.
The time occupants have to escape varies according to the size of the fire or the cause of the collapse. In tests done on structures containing a small fire, occupants could move freely within the structure while the fire was still burning. Larger fires caused a quick collapse, which presents a hazard because the escape-to-collapse time is shortened. To delay this deflation and prevent the fabric from collapsing to the ground, provide some additional means of support to keep the fabric level with the height of the door.
Air-supported structures, although very profitable for the manufacturer and property owner, pose a great danger if air pressure is lost. The chances that civilians will become trapped varies with the cause of the collapse. Even with manufacturer warranties lasting 20 years, these structures can fail for a multitude of reasons. Being aware of their designs and limitations can help you make more educated decisions in a catastrophe.
The incident commander will need to determine whether an interior or an exterior attack is appropriate, based on the life hazard present, the operation of the fans, the weather conditions, and the size of the fire. Preplan air-supported structures in your district, including the location of the primary and secondary fans and the utility shutoffs. The facility engineer can provide a wealth of knowledge and offer guidance during an emergency. Conduct drills in these facilities to familiarize your department with their size, layout, and occupancy potential.
References
1. International Building Code. (2018). Chapter 31, Special Construction. https://bit.ly/2KUXFCa.
2. Custer, Richard LP. “Test Burn and Failure Mode Analysis of an Air-Supported Structure.” Fire Technology, February 1972, 8(1), 19-23. https://bit.ly/2s5yvtW.
3. Malhotra, HL. “The Behaviour of Polymers Used in Building Construction.” Fire Research Note No. 1071, The Fire Research Station, July 1977. https://bit.ly/2s34b31.
4. Hopkinson, JS. “Fire Tests on Air-supported Structure.” Fire Research Note No. 955, The Fire Research Station, December 1972. https://bit.ly/2IIQaSg.
5. Lloyd, Aaron T. “What Happens When the Bubble Bursts? Why Sports Teams Must Learn From the Collapse of the Dallas Cowboys’ Practice Facility.” Willamette Sports Law Journal, Fall 2010, 46-60. https://bit.ly/2s70Z5N.
STEPHEN RHINE is a 15-year veteran of and a lieutenant with the Fire Department of New York (FDNY), assigned to Engine 287; a member of the FDNY All Hazards Type 2 Incident Management Team; and a 24-year member of the Roslyn Highlands (NY) Volunteer Fire Department. He is a New York State-certified fire instructor and fire officer and a graduate of the West Point Counterterrorism Leadership Graduate Program.