Building fires are most often controlled by the direct intervention of responding firefighters; at other times, fire sprinkler systems may suppress the fire. However, sprinkler systems may encounter water delivery limitations and logistical obstacles may hinder firefighting efforts—e.g., delayed access to a burning high-rise building’s upper floors. Hence, a fire may become uncontrolled and we must rely on the structure’s intrinsic safety to protect building occupants and firefighters.
When uncontrolled fire heats structural systems, they experience thermally induced forces/rotations for which they are not generally designed. We will examine how structures are typically protected from fire; the recent push to explicitly design structures for fire in the same way that they are designed to withstand earthquakes, hurricanes, and other hazards; and the firefighting community’s critical role in these efforts.
Structural Fire Safety
Most fire sprinkler systems are generally intended to control a single fire that involves ordinary combustibles. A fire sprinkler system’s hydraulic capacity is typically only designed for a small cluster of sprinkler heads (five to 10) at a single location within a building.1 Accordingly, a fire sprinkler system’s effectiveness decreases dramatically as the number of sprinkler heads activated exceeds its designed capacity (e.g., 10 or more sprinkler heads).2 Consequently, fire sprinkler systems may not be effective at controlling large/intense fires or fires occurring in multiple locations simultaneously. Such fires pose significant challenges to firefighters, and the reliability of structures under such circumstances is critical and often taken for granted by the design community. This is despite the fact that the code permits reductions in fireproofing to steel if a fire sprinkler system is installed as well as reductions for other superficial aspects (e.g., restraint classification3) that have been successfully lobbied over time. Historically, the required level of fireproofing in buildings has generally declined over the past century. However, the importance of structural fire safety to protect building occupants and firefighters remains constant.
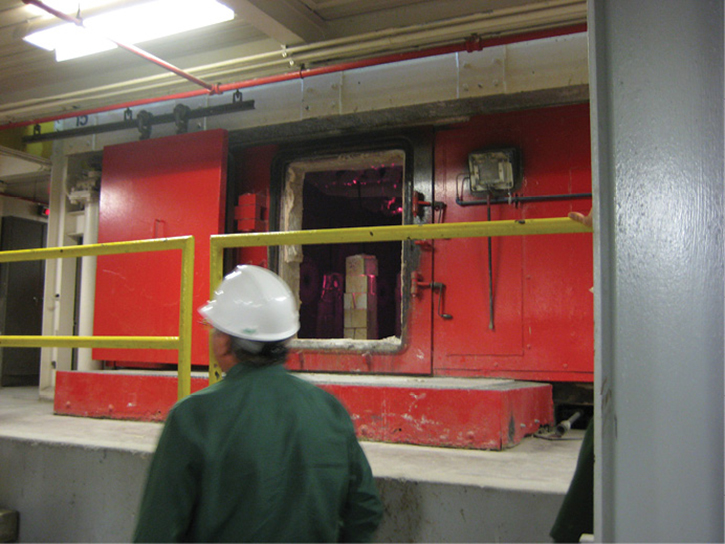
(1) Photo by Kevin LaMalva.
Unquantified Structural Fire Safety
Traditionally, fireproofing is prescribed for structures after they have been designed for other hazards such as earthquakes and hurricanes. Fireproofing is qualified by standard furnace testing (e.g., ASTM E-119, Standard Test Methods for Fire Tests of Building Construction and Materials), which has remained relatively unchanged for the past century (photo 1). Furnace testing excludes consideration of critical structural connections, structural system response, and actual fire behavior. Also, the size limitation of a test furnace restricts structural component spans to typically no greater than 17 feet, which further trivializes its representation of actual structural systems (Figure 1).
Figure 1. Test Furnace vs. Actual Construction

Figure by Kevin LaMalva.
Finally, the near-artisan application of fireproofing to test mock-ups for furnace testing qualification (photo 2) may not resemble actual installed conditions (photo 3). Because of these limitations, structural fire safety advocates have recognized for decades that there is no correlation between a successful furnace test and the performance of a corresponding structural system under actual fire conditions.4
Code-prescribed fireproofing helps to reduce the heating of individual structural elements (e.g., a steel beam or a column); however, the actual anticipated structural system performance under fire exposure is neither confirmed nor quantified.5 For instance, the stability of structural connections as structural elements expand/contract under heating/cooling is not considered. This is despite the fact that forces and rotations resulting from restrained thermal expansion/contraction often dominate the overall structural system performance, even more so than the temperature of individual members (on which most furnace test acceptance criteria are based). In other words, structures are traditionally not engineered to withstand fire effects but rather are blanketed with fireproofing with the intent of such. Consequently, a building’s vulnerability to structural collapse from uncontrolled fire varies from building to building and is subject to coincidental structural engineering design decisions made for other hazards.6

(2) Photo by Kevin LaMalva.

(3) Photo by Glenn Corbett.
Standard furnace testing used to qualify fireproofing typically permits the temperature of structural elements to reach as high as 1,300°F and still pass. This is despite the fact that the forces and rotations induced in structures can become very significant at temperatures as low as 300°F. Considering the seismic design of structures, this would be analogous to prescribing base isolators (i.e., structural elements at the base of a building that allow for a building’s substructure and superstructure to move independently of each other during an earthquake) for a building to reduce, but not eliminate, earthquake-induced forces with no analysis or understanding of the structure’s performance to such forces (Figure 2). Considering the wind design of structures, this would be analogous to encapsulating buildings with wind shields to reduce, but not eliminate, hurricane-induced pressures without any analysis or understanding of the structure’s performance under such pressures (Figure 3). Rather, structural engineers routinely assess the structure’s intrinsic ability to withstand such forces, often necessitating the addition of lateral bracing and/or structural connection upgrades. Why should there be a different standard of care for fire-induced forces? At the same time, the traditional method of protecting structures from fire may not be keeping pace and properly accounting for new construction trends (e.g., cross-laminated timber structures and super high-rise buildings), which may not have been envisioned a century ago. Whereas many industry stakeholders remain complicit and are inclined to extrapolate archaic methods to meet modern design/construction demands, the firefighting community must continue to advocate for structures that are intrinsically safe to fire.
Figure 2. Fictitious Seismic Design
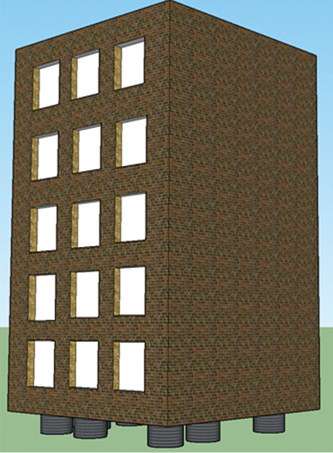
Figure 3. Fictitious Wind Design

Figures 2, 3 by Kevin LaMalva.
Unregulated Structural Fire Safety
In response to new and rapidly evolving building construction trends, designers have increasingly pursued alternatives to prescriptive fireproofing requirements. However, the United States has long lacked an industry consensus on what is permitted and satisfactory in this respect. Specifically, International Building Code (IBC) 7 703.3(5) states that an alternative approach may be used; however, the code provides no specific requirements, guidance, or bounds. Consequently, alternative approaches for structural fire protection often have a wide variation in engineering rigor and conservatism, with most tending toward less rigor/conservatism.8 For instance, a designer may seek to justify the removal of fireproofing from steel structures based solely on fire modeling (Figure 4) with no appreciable structural system analysis. Unfortunately, possible inherent defects or system vulnerabilities associated with this type of poor practice would not be uncovered unless an uncontrolled fire event tests the true adequacy of such designs.9 Hence, the continued advocacy of the firefighting community for involvement in these endeavors can help guard against poor practices and unsafe designs.
Figure 4. Fire Modeling

Figure by National Institute of Standards and Technology.
Quantification and Regulation Are Needed
Although somewhat uncommon, severe structural damage and collapses of engineered buildings resulting from fire [e.g., One Meridian Plaza,10 World Trade Center (WTC) 1 and 2 buildings,11 WTC 5 building,12 WTC 7 building,13 Windsor Tower,14 Delft Technical University building,15 Tour d’Ivoire,16 and others] have highlighted the need for the explicit design of structures for fire. Notably, standard furnace testing results would not have anticipated the collapse mechanisms these events exhibited (e.g., structural connection failures).17
As early as 1967, the following was declared regarding the inability of furnace testing to capture realistic structural system behaviors18:
If we attempt to develop the fire endurance of a construction system in actual buildings under fire conditions we would not obtain a single-valued answer, but rather we would have to measure a range of performance levels depending upon methods of structural framing existing in a single building as well as the methods of structural framing of any and all buildings into which the construction system under consideration could be incorporated ….
More recently, the National Institute of Standards and Technology (NIST) recommends the following within its WTC investigative report19:
NIST recommends the development of: (1) performance-based standards and code provisions, as an alternative to current prescriptive design methods, to enable the design and retrofit of structures to resist real building fire conditions, including their ability to achieve the performance objective of burnout without structural or local floor collapse.
The deep entrenchment and significance of standard furnace testing within all aspects of structural fire safety is perhaps because of the evolution of the ASTM committee that maintains the contemporary ASTM E11920 furnace test standard. When ASTM as a body began, changes to standards were relatively straightforward and transparent within the public record (all meeting minutes can be readily obtained in historical transactions). In the early days, the committee membership overseeing the ASTM E119 standard consisted of fewer than 20 representatives, primarily of professional societal and insurance backgrounds. In general, few material manufacturers were represented on the committee when it began. In fact, at its formation, those from the material industries in association with the society were largely from the concrete industry and expressed that the committee could be at risk of being regarded as a trade organization.21 For example, the following was stated at the committee’s first attempt to standardize furnace testing in 190722:
… The American Society for Testing Materials with its committees stands for fair play for all materials having common use and a common purpose, and we do not want the specifications that go to the public to be questioned as being influenced by cement men, by concrete men, or by men in any other form of industry or trade. This is a broad scientific body, and not a trade organization.
As the standard furnace test became enforced and listings began to be codified, the committee’s makeup evolved and changed. Various material representatives from across the construction industry joined the committee with what appears to be commercial interests as a key motivator. For example, the following was proclaimed at the time23:
Manufacturers (need to) know the resistance periods of their materials and increase them if possible. [Financial] Competition must be met on the basis of performance in tests.
Presently, materials industries are well represented in the ASTM committee that maintains the ASTM E119 standard and there appears to be very little motivation for change.
Historically, structural engineers have neither been directed or expected to consider the fire safety of structures. Instead, the traditional protection method is employed by any one of a variety of design professionals, most often by architects. However, a fast-growing segment in the U.S. structural engineering community has become more assertive that their involvement and assimilation into structural fire safety endeavors is warranted.24 As structural fire designs conducted in Europe over the past decade have shown, structural engineers have the expertise to explicitly design structures to adequately endure uncontrolled fires. For instance, targeted upgrades to slab reinforcement and structural connections can be readily implemented to allow for the safe deflection of floors under heating without collapse. Also, columns along a building’s periphery can be strengthened to withstand floor expansion resulting from fire exposure without catastrophic buckling. Such measures can dramatically raise a structure’s intrinsic fire safety compared to fireproofing the members, especially considering the dramatic variability commonly observed in the quality/condition of installed fireproofing. Above all, these critical design considerations are entirely outside the predictive capabilities of standard furnace testing used to qualify fireproofing. Hence, the alignment of structural system performance expectations during uncontrolled fire conditions with the needs of the firefighting community is key to successful reform.
Quantified and Regulated Structural Fire Safety
The American Society of Civil Engineers/Structural Engineering Institute’s (ASCE/SEI’s) recent efforts have culminated in an industry consensus for the explicit design of structures to safely withstand uncontrolled fire exposure, which is slated to be addressed in the coming round of code changes.25 Specifically, it is proposed to delete the vague and often exploited language of IBC 703.3(5) and replace it with a direct reference to ASCE/SEI 726, which, for the first time, now contains provisions that pertain to the explicit design of structures for fire conditions. Specifically, ASCE/SEI 7 Section 1.3.7 stipulates that structural fire protection design shall be conducted in accordance with the prescriptive requirements of the applicable building code (with no deviation) or in accordance with the structural fire design requirements of ASCE/SEI 7 Appendix E per discretion of the building authority. Supporting the provisions of Appendix E, the new and first-of-its-kind ASCE/SEI Manual of Practice (MOP) No. 138 (Structural Fire Engineering) 27 has been developed and published, which provides specific guidance for conducting structural analyses for fire conditions. Unlike traditional protection methods, the ASCE/SEI 7 Appendix E method requires the responsible charge of a structural engineer in all cases.28 Also, unlike the traditional method, the ASCE/SEI 7 Appendix E method requires the explicit confirmation of structural system performance expectations. For instance, the consequences of increased/delayed occupant evacuation,29 reliance on building refuge areas, and all other performance expectations of the building authority must be properly considered. By design, ASCE/SEI 7 Appendix E and ASCE/SEI MOP No. 138 provide building authorities with a potent set of tools to comprehensively evaluate alternative methods for structural fire protection. Hence, it is important for the firefighting community to engage with building authorities in these cases so that their needs can also be properly met.
Retrospect
We should not take the performance of structural systems during uncontrolled fire exposure lightly. Accordingly, the firefighting community must continue to advocate for structural fire safety and be empowered to expect more from the design community in this respect. Recent standards from ASCE/SEI (with prospective code adoption) are a step in the right direction toward more intrinsically safe structures to fire. Indeed, structural fire safety is more important than ever given the rapidly evolving design/construction landscape, which is perhaps outpacing traditional and century-old protection methods. Moving forward, it is envisioned that the design of structures will consider earth (seismic motions), wind (hurricane pressures), and fire (elevated temperatures and thermal expansion/contraction).30 Promisingly, the conditions for reform are improving as industry advancements and education in structural fire safety continue to accelerate in the United States.31
References
1. NFPA 13, Standard for the Installation of Sprinkler Systems, National Fire Protection Association, Quincy, Massachusetts, 2016.
2. Hall, J. “U.S. Experience with Sprinklers and Other Automatic Fire Extinguishing Equipment,” National Fire Protection Association, Quincy, Massachusetts, 2010.
3. LaMalva, K.; Gernay, T.; Bisby, L.; Torero, J.; Solomon, R.; Gales, J.; Hantouche, E.; Morovat, A.; Jones, C. (2020) “Rectification of Restrained vs. Unrestrained,” Fire & Materials Journal, Special Issue: 100 Years of Fire Resistance Design, Vol. 44, No. 1, <https://doi.org/10.1002/fam.2771>.
4. Law, M. (1981) “Designing Fire Safety for Steel–Recent Work,” ASCE Spring Convention, American Society of Civil Engineers, Reston, Virginia, USA & Pettersson, O. (1975) “The Connection Between a Real Fire Exposure and the Heated Conditions According to Standard Fire Resistance Tests-with Special Application to Steel Structures,” Bulletin 39, Lund Institute of Technology, Lund, Sweden.
5. UL Fire Resistance Directory, Underwriters Laboratories, Northbrook, IL.
6. LaMalva, K. (2018) “The Time Is Right for Structural Engineers to Embrace Structural Fire Protection,” ASCE/SEI Update, American Society of Civil Engineers: Structural Engineering Institute, Reston, VA.
7. International Building Code. (2018) International Code Council, Washington, DC.
8. Jonsson, J. (2019) “Are You A Competent Practitioner,” SFPE Europe, Q1, Issue 13, Society of Fire Protection Engineers, Bethesda, MD.
9. Jonsson, J. (2019) “SFPE Core Competencies: Why Do We Need Them,” Fire Protection Engineering Magazine, Q1, Society of Fire Protection Engineers, Bethesda, MD.
10. Eisner, H.; Manning, W. (August 1991) “One Meridian Plaza Fire,” Fire Engineering.
11. Gross, J., et. al. (2005) “Structural Fire Response and Probable Collapse Sequence of the World Trade Center Towers,” NIST NCSTAR 1-6, National Institute of Standards and Technology, Gaithersburg, MD.
12. LaMalva, K.; Barnett, J.; Dusenberry, D. (2009) “Failure Analysis of the World Trade Center 5 Building,” Journal of Fire Protection Engineering, Vol. 19, No. 4.
13. McAllister, T., et. al. (2008) “Federal Building and Fire Safety Investigation of the World Trade Center Disaster: Structural Fire Response and Probable Collapse Sequence of World Trade Center Building 7,” NIST NCSTAR 1–9, National Institute of Standards and Technology, Gaithersburg, MD.
14. Fletcher I., et. al. (2006) “Performance of Concrete in Fire: Review of the State of the Art, with a Case Study of the Windsor Tower Fire,” Proceedings of the 4th International Workshop for Structures in Fire, Vol. 2.
15. Meacham, B., et. al. (2010) “Fire and Collapse, Faculty of Architecture Building, Delft University of Technology: Data Collection and Preliminary Analyses,” CMMI Research and Innovation Conference, National Science Foundation.
16. Burnier, O. (2011) Reconstitution de l’incendie de deux voitures dans le parking de la Tour d’Ivoire à Montreux, le 9 décembre 2010, Travail de diplôme, heig-vd ed., Yverdon-les-Bains.
17. LaMalva, K.J. (editor), ASCE/SEI Manual of Practice No. 138: Structural Fire Engineering (2018), American Society of Civil Engineers: Structural Engineering Institute, Reston, VA.
18. Bletzacker, R.W. “Fire Resistance of Protected Steel Beam Floor and Roof Assemblies as Affected by Structural Restraint,” Symposium on Fire Test Methods-Restraint & Smoke 1966, ASTM STP 422, American Society for Testing and Materials, 1967, 63-90.
19. Shyam-Sunder, S., et. al. (2005) “Federal Building and Fire Safety Investigation of the World Trade Center Disaster,” NIST NCSTAR 1, National Institute of Standards and Technology, Gaithersburg, MD.
20. ASTM E119. (2016) E119-16a Standard Test Methods for Fire Tests of Building Construction and Materials, ASTM International, West Conshohocken, PA.
21. LaMalva, K.; Gernay, T.; Bisby, L.; Torero, J.; Solomon, R.; Gales, J.; Hantouche, E.; Morovat, A.; Jones, C. (2020) “Rectification of Restrained vs. Unrestrained,” Fire & Materials Journal, Special Issue: 100 Years of Fire Resistance Design, Vol. 44, No. 1, <https://doi.org/10.1002/fam.2771>.
22. ASTM Proceedings, Volume 7, Committee P-Fire Minutes, 179-180, 1907.
23. Foster, H. (February 1929) “Significance of the Standard Fire Test to Heavy Clay Products Manufacture,” Annual Meeting, American Ceramic Society, February 1929, https://bit.ly/3myLuhr
24. Ellis, A. (March 2019) “Unleashing the Profession: How Performance-Based Design Will Shape Our Future,” STRUCTURE Magazine.
25. LaMalva, K.; Wittasek, N. (May 18, 2020) “New Standardization for Structural Fire Protection Variances,” ICC Building Safety Journal, International Code Council, Washington, DC. https://bit.ly/3kRshH6.
26. ASCE/SEI 7: Minimum Design Loads and Associated Criteria for Buildings and Other Structures (2016), American Society of Civil Engineers: Structural Engineering Institute, Reston, VA.
27. LaMalva, K.J. (editor), ASCE/SEI Manual of Practice No. 138: Structural Fire Engineering (2018), American Society of Civil Engineers: Structural Engineering Institute, Reston, VA.
28. SFPE Recommended Minimum Technical Core Competencies for the Practice of Fire Protection Engineering, Society of Fire Protection Engineers (2018), Bethesda, MD.
29. SFPE/ICC Engineering Guide: Fire Safety for Very Tall Buildings (2013), Society of Fire Protection Engineers, International Code Council, Washington, DC.
30. Cocke, D. (2019) “SEI Annual Report: Performance-Based Design,” American Society of Civil Engineers: Structural Engineering Institute, Reston, VA.
31. CPF Grant #04-18. “Advancing Performance-Based Structural Fire Engineering Design in the U.S. through Exemplar Procedural Guidance,” Charles Pankow Foundation, Vancouver, WA, 2018. https://bit.ly/3myNVk5.
KEVIN LaMALVA, P.E., is a principal fire consultant with Warringtonfire and has dual registrations in fire protection engineering and civil engineering. He is past chair (2013-2018) of the ASCE/SEI Fire Protection Committee and is a member of numerous industry committees that conduct research and develop standards for structural fire safety.
GLENN CORBETT, P.E., is a former assistant chief of the Waldwick (NJ) Fire Department, an associate professor of fire science at John Jay College of Criminal Justice in New York City, and a technical editor for Fire Engineering. He served on the Federal Advisory Committee of the National Construction Safety Team and is a member of the Fire Code Advisory Council for New Jersey. He is the coauthor of the late Francis L. Brannigan’s Building Construction for the Fire Service, 6th Edition, editor of Fire Engineering’s Handbook for Firefighter I and II, and an FDIC executive advisory board member.