By Richard C. Beaulieu
As the public’s personal one-stop shop for the multitude of emergency situations that can arise in the world, the fire service must maintain a vast collection of knowledge, skills, abilities, and equipment that we use every day to help our citizens. Firefighters use some equipment daily and are proficient in using it, while other items get locked away in the vault of our minds and our trucks to be pulled out in those rarest of rare situations.
Experience has taught us that whether something is used often or only once in a career, ongoing training is crucial to the success of our operations. Just because we use our defibrillator a dozen times a day does not mean we can skip a class on 12-leads and cardioversion. On the flip side, we need to ensure that a blanket of rust does not form on our technical rescue skills. Even though many of us use our multigas meters daily for gas leaks and carbon monoxide (CO) calls, there’s a lot more to these instruments that is worth reviewing in the context of some actual incidents and hypotheticals.
RELATED FIREFIGHTER TRAINING
- Hazmat: Detection and Metering for Civilian Rescue While Wearing Turnout Gear
- Kevin Yoos: The Multigas Meter
- Hazmat Size-Up: A New Model for Rapid Decision-Making
- Hazmat Survival Tips: Interpreting Readings from Atmospheric Monitors
The multigas meter is the ultimate symbol of daily hazmat response. When we get dispatched to a daily routine hazmat call such as a CO alarm sounding, our Dispatch, Rescue/Public Protective Actions, Environment, Container, Chemical (DRECC) size-up is fairly straightforward. A heavy rescue is dispatched to a residence in the winter for a CO alarm. The DRECC size-up is as follows:
Dispatch: 101 West Street for a CO alarm activation.
Rescue/Public Protective Actions: On arrival, residents may need evacuation.
Environment: Indoors at a residential home during wintertime.
Container: Possible malfunction of the heating system, the stove, or the water heater.
Chemical: Reported as CO from a residential detector.
With aging heating systems, it is not suspicious or uncommon to receive a plethora of CO alarm activations in the winter in New England, so it raises no red flags for a company officer. The fire service knows and understands the substance as a toxic flammable gas that is colorless, odorless, and lighter than air. The compound is a chemical asphyxiant that replaces oxygen (O2)in the hemoglobin.
The officer en route is readying the multigas meter. Many times, the CO levels are zero and there is a detector battery or power issue that the homeowner must resolve. Sometimes, the dispatch involves reported symptoms such as headaches and dizziness; the response needs should include additional companies and medical units.
Department policies and procedures will typically have some sort of action level that requires companies to don self-contained breathing apparatus (SCBA) if levels come close to or exceed the National Institute for Occupational Safety and Health (NIOSH) recommended exposure limit (REL) or the Occupational Safety and Health Administration (OSHA) permissible exposure limit (PEL). In this case, the company enters the scene and the O2 sensor is reading a normal atmosphere of 20.9%; readings are 60 parts per million (ppm) on the first floor.
The homeowners meet the company on the front lawn and report that they recently had work done on their heating system. The company dons SCBA on their initial reading and investigates the basement, where they find readings of 150 ppm near the heating system.
They shut down the heating system and ventilate the home, and now their readings are at zero. The homeowners will need to find shelter for the evening and contact the company that worked on their heating system.
This scenario will likely sound familiar to all fire service members. Often, on medical calls, the caller’s symptoms end up as the result of an exposure to a hazardous material.
As an officer on a rescue (medical unit), I had been treating a patient in the back of the truck when suddenly my SpCO reading pops up on the defibrillator with a SpCO of 12%; the person explained that he was just smoking cigarettes, and this makes sense. I have had high SpCO readings in people who had recently smoked a hookah as well.
On other occasions, patients will have a high SpCO with no reasonable explanation. A company will then check the home for CO to ensure the safety of the rest of the residents.
We had a call several years ago for a person complaining of dizziness. He was working in a basement on a damaged foundation and he began feeling dizzy and faint. He went outside and felt better so he went back into the basement to work. In the basement, his symptoms returned and he called for an emergency medical services (EMS) response. After hearing the story, the engine company metered the basement and found an O2-deficient atmosphere. There was no discernible reason for this atmosphere to be O2 deficient at first. The next step was to confirm this finding using a secondary meter and then a detector tube. Both meters indicated an O2-deficient atmosphere, and a detector tube specific to O2 backed up what the meter was showing.
Our deputy chief of hazmat was called to the scene and, after doing some research, used a detector tube for carbon dioxide (CO2). This tube revealed that CO2 was displacing the O2 in the basement; this was the cause of the workman’s symptoms. Further research showed that sometimes CO2 can leach through soil from decaying matter and with a damaged foundation it had found its way into the basement of this home.
On another occasion—ironically while officers were teaching hazmat to a recruit class—the fire station’s CO alarms started going off upstairs in the administrative offices, in our training room, and in dispatch. The heating system was not running because the weather was relatively warm. We walked the building with our multigas meters and our CO sensors reading 30 to 40 ppm. We then took out detector tubes and tested the dispatch room, the administrative offices, and the training room; we had zero ppm for CO.
We needed to figure out exactly why the meters designed to detect CO were in alarm. Some sensors have cross-sensitivities; they will activate in the presence of something other than the intended material. On further investigation, we learned that the maintenance division on the first floor had accidentally left their acetylene tank slightly cracked open and everything made sense. Acetylene is a known cross-sensitive material to CO sensors; since it is lighter than air, it found its way to the upstairs offices. We shut off the tank, we ventilated the building, the station detectors deactivated, and our meters had zero readings.
It is paramount for the safety of the public and firefighters responsible for everyday hazmat incidents to fully understand the meters’ uses and limitations. Knowing the type of technology in the meter is not as important as understanding its calibration gas, how it affects the readings we see in the field, applying correction factors, the action levels your department policies and procedures require, the meter’s reading limits, its performance under certain conditions, and its cross-sensitivities and to confirm all readings with multiple technology. This explanation below is specific to the brand of gas detectors our department uses.
Calibration Gas and How It Relates to Readings
Gas detector sensors are calibrated with the gases we use today for many historic reasons, but it is most important to know how this affects the instrument’s response. Our particular meters typically can measure O2; the lower explosive limit (LEL); and volatile organic compounds (VOC), which are the representation of the photo ionization detector (PID), CO, and hydrogen sulfide (H2S). All of our fire apparatus and our battalion chiefs are equipped with these meters.
The H2S sensors on some meters have been replaced with hydrogen cyanide (HCN) sensors for use during overhaul after fires. Gas detection companies have begun offering multigas meters with four, five, and six sensor slots (photo 1). We recently acquired a six-gas meter that equips sensors for H2S and HCN simultaneously.
Advances in technology have allowed meters to be tracked using GPS and transmit data back to a computer in the command post in real time. Some even have man-down features that will either send text message distress signals to a designated phone list or will contact the user directly through the meter. Additionally, some newer meters can read gamma radiation (photo 2). If using one of these meters at an incident, remember that the pump will run for 50 seconds measuring the air and will stop for 10 seconds to read gamma radiation. For 10 seconds, you could potentially be walking into a higher concentration of a gas without seeing the upward trend until the pump resumes measuring air.
Always remember to meter the floor, the mid-level, and the ceiling of a room if a gas is unknown because of different vapor densities. Responders should know the vapor density of the most common chemicals encountered. CO, natural gas, and HCN are lighter than air, requiring metering at the upper levels of a room or structure. H2S, propane gas, and chlorine gas are heavier than air and require metering at lower levels. This all goes back to our full assessment of what should and should not be present at a given scene after our size-up.
Sensors designed to read specific chemicals are calibrated to that gas. Sensors that are designed to read a multitude of chemicals have one specific calibration gas and will have a direct read when you know for a fact that you are measuring that gas in an atmosphere. All other substances require correction factors if the sensor can even detect them.
Most multigas meters will prompt the user during its start-up procedures for a “fresh air calibration” or the user can access this option in the calibration section of the menu. Other instruments have specific buttons to perform this operation at will. This allows the meter to zero out the sensors. Ensure that the air is actually fresh before doing this calibration, or readings will be skewed. For example, do not perform a “fresh air calibration” near the truck’s exhaust.

(1) Photos by author.
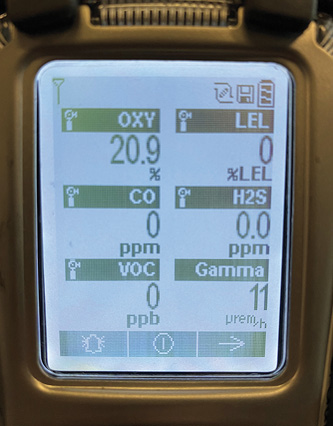
(2)
The LEL and PID sensors are the most important. The LEL sensor is calibrated to methane gas, the predominant gas found in natural gas. We all respond to natural gas emergencies regularly since the gas is used for cooking and heating. Therefore, our LEL sensor being calibrated to methane gas allows for a direct read at natural gas emergencies. It is imperative to remember that the LEL sensor reads in percentage. This sensor is calibrated to the LEL of methane, which is 5% or 50,000 ppm.
In an atmosphere where the LEL sensor is reading zero, this does not mean that nothing is there. Since the sensor displays in whole numbers only, you would not find 1% on the meter on the methane gas setting until it reaches 500 ppm, or 1% of 50,000 ppm. Gases can simultaneously be flammable and toxic; often, toxicity and damage to the body occur at levels significantly lower than the LELs. Hence, 450 ppm of a substance could be lethal while the LEL sensor is reading zero.
Consider anhydrous ammonia. It would take 150,000 ppm of this gas in a confined or enclosed atmosphere to ignite, but its immediately dangerous to life or health (IDLH) level is 300 ppm!
Furthermore, do not confuse the readings on your LEL sensor when responding to natural gas emergencies with what the gas company is getting on its meter. The gas company’s meter is not set to the LEL of methane.
For example, if a residence has 50,000 ppm of natural gas present, while our meter would be reading 100%, the gas company’s meter would be reading 5%. This is purposely done that way because we respond to a multitude of hazmat incidents and need to safely measure a lot more than just natural gas. It is paramount to our safety to know when we are approaching an LEL.
The PID reads in ppm. Some of the newest technology will start off reading in parts per billion (ppb), as can be seen in photo 3 under the VOC reading, and then automatically switches to ppm as the concentration increases. The PID is often represented on the screen of the meter as VOC—this is misleading because the sensor can detect inorganic compounds as well. This sensor is calibrated with isobutylene and can detect as little as 0.1 ppm. This sensor is for use when toxicity is a concern and we must be able to read the lowest possible concentrations.
For example, benzene is commonly found with petroleum products, has a NIOSH REL of 0.1 ppm, and is a known carcinogen. A detailed list of what these sensors are capable of measuring is available online but should be printed out and kept in a binder as well. This becomes important for applying correction factors or knowing whether the meter will even detect a substance.
To reiterate, just because the meter is not reading a substance does not mean that nothing is present! Responders must rely on their size-up of the situation, the type/occupancy of the incident, what chemicals should be there and what should not, and the symptoms of those exposed to drive their incident action plan.
Correction Factors and What the Sensors Can Measure
Technical notes from your detector’s manufacturer are available online; you will need to use them when the LEL and PID sensors are reading known substances. You will need additional technology to further classify any unknown substances that produce meter readings; the responding hazmat response team or hazmat technicians are responsible for this.
Also, understand that the PID sensor has a specific lamp. These sensors ionize a substance using light; different lamps that are available deliver different amounts of energy. The different lamps as listed in our detector manufacturer’s technical note for PID sensors are 9.8 electron Volts (eV), 10.6 eV, and 11.7 eV; there is a list of specific chemicals that each lamp is able to detect. When researching a chemical, if it has an ionization potential of 9.67 eV, then any of the lamps will be able to measure (ionize) the compound; if the ionization potential is greater than 11.7, none of the lamps will be able to read that substance.
In our department we use a 10.6 eV lamp for our PID sensor, so the sensor is unable to see chlorine gas, which has an ionization potential of 11.48 eV. However, we can measure anhydrous ammonia (NH3), but for an accurate reading, we need to use the technical note to apply a correction factor. If we go into an atmosphere of NH3 and our VOC reading is 100 ppm, the listed correction factor is 10.9, which would provide a corrected reading of 1,090 ppm of ammonia.

(3)
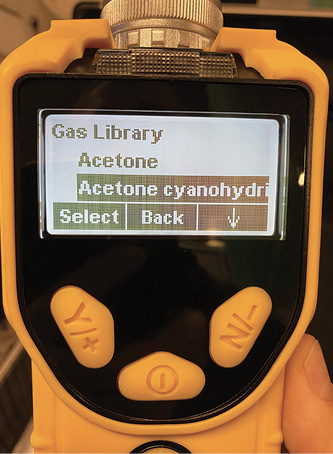
(4)
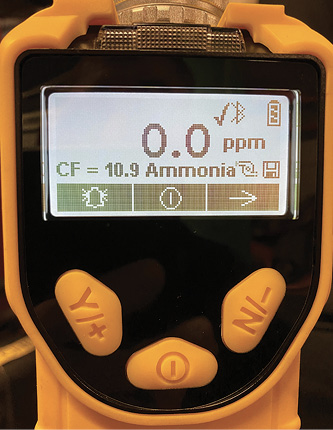
(5)
In photos 4 and 5, the detector is not a multigas meter; it is a dedicated PID with a library, so if the chemical is known and it is in the library, you can change to that chemical and then correction factors are not needed.
The same is true for the LEL sensor where we would check the list in the manufacturer’s LEL technical note to see if the chemical we are trying to measure is present and then multiply our reading by the correction factor. For ammonia, if the LEL sensor was reading 10%, the correction factor is 0.9, and after multiplying the reading by the correction factor, we are actually at 9% of the LEL of ammonia.
Action Levels
Action levels determine how we will respond based on the readings our meters provide and how we interpret the readings and data. Depending on the material, regulations, consensus standards, or your agency standard operating procedures (SOPs) will determine your action levels. Industry and emergency response use action levels in the form of exposure limits, which is the concentration the industrial hygiene community has set to determine the level of personal protective equipment (PPE) needed in the presence of a given substance. Below the exposure limits, no PPE is required.
For example, for carbon monoxide, NIOSH has set the REL for CO at 35 ppm; at or below this level, no PPE is required for an eight-hour workday, 40-hour-per-week exposure. This is our department’s CO response SOP: At or below 35 ppm, we do not need to be on air, but above it responders must don a face piece and go on air. The only people who should be operating above the exposure limits are those trained in metering and wearing chemical protective clothing and respiratory protection. If a given compound exceeds concentrations above IDLH levels, only qualified personnel should be operating in this environment.
HCN is another substance for which we have an action level SOP. After suppressing the fire, overhauling, and ventilating the space, we also meter it; the HCN level must be zero for anybody to be in the structure without SCBA on air. We are revising and expanding this policy because of the multitude of dangerous compounds structure fires create. The best policy to maximize long-term health and well-being in the fire service is to use respiratory protection whenever operating within a toxic atmosphere.
In addition to CO and HCN, several toxic, carcinogenic, and corrosive chemicals may be created in a burned structure. Some toxic gases are also corrosive including ammonia, hydrogen fluoride, hydrogen chloride, and formic acid. In a hazmat incident, the presence of these gases would require Level A protection, reinforcing the point that wearing respiratory protection during overhaul is imperative for firefighters’ health and safety.
Some additional toxic gases present are also carcinogenic, including acetaldehyde, acrolein, acrylonitrile, benzene, formaldehyde, glutaraldehyde, isocyanates, naphthalene, nitrogen oxides, sulfur dioxide, toluene, and vinyl chloride. Many more compounds certainly exist; this list of chemicals is from this detector manufacturer’s technical note for its overhaul library. This device offers different libraries based on the operation: weapons of mass destruction, toxic industrial chemicals, clandestine labs, or overhaul. When it detects a substance in the overhaul library, it will go into alarm and visually alert users to “mask up” if it detects any of the chemicals listed above at their time-weighted average (TWA) levels. Although the meter will not visually identify the particular compound, it will tell the user to “mask up” if it detects a library chemical; if the substance detected is not in the library, the display will read “chemical detected” (photo 6).
Decontamination
Departments should adopt policies to treat structure and vehicle fires like hazmat scenes and be aggressive about metering, ventilation, and respiratory protection in their aftermath. Decontamination is another often-overlooked aspect of postfire operations. My department has improved on decontamination after a fire operation in the past few years. Initially, you should perform decontamination at the scene and then more thoroughly back in quarters using extractors to clean gear. Setting up and performing decontamination at a hazmat scene are built into the OSHA standard so it always performed. The fire service needs a cultural shift toward mandatory decontamination after exposure to products of combustion. It is vital to having a healthy career and a lengthy retirement to protect ourselves from chemical exposure whether at a hazmat incident or a structure/vehicle fire.
O2
When metering a space, the O2 concentration must be between 19.5% and 23.5%; concentrations below 19.5% or above 23.5% are considered IDLH atmospheres. O2 is usually 20.9% in most spaces. O2 represents one-fifth of the gases of the air in our atmosphere. Why is this important? If we are metering a space and the reading starts at 20.9% O2 and, as we continue into a facility, the O2 rapidly declines to 19.9%, it means that 50,000 ppm of a gas has displaced that air—including its O2. We must then try to figure out if and why any gases would be present in this location that displace O2 such as CO2.
Although responders can use SCBA in an O2-deficient atmosphere, protection from an O2-enriched atmosphere is more challenging. In that case, ventilate the space and reassess how the O2-enriched environment was created. In the O2-deficient atmosphere resulting from CO2 displacing the O2 in the residential basement discussed above, we protected ourselves with SCBA while we used multiple technologies to meter that space.
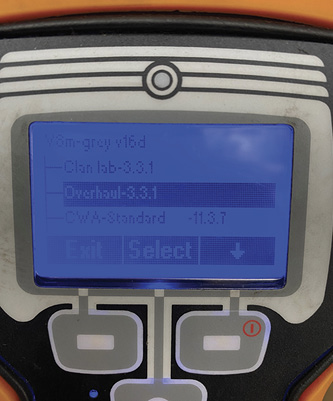
(6)
LEL Sensor
An important action level to remember concerns the LEL sensor. The LEL sensor’s action level is 10%. If it is a natural gas emergency and we know that our meter is calibrated to methane, then we are not in danger at 10% and we could continue with locating the source of the leak and trying to mitigate it while ventilating. The manufacturer’s technical note for the LEL sensor includes a long list of compounds that it can detect. When dealing with an unknown atmosphere, our action level is 10% because below 10% we can be sure that we are safe to continue metering, but at 10% we must stop, back out, ventilate, and reassess. Some of the chemicals that the LEL sensor can detect have correction factors above 5; if we pushed the limits to 20%, we could be at or above the substance’s LEL and place ourselves in extreme danger.
Depending on the scenario and the associated risks, the incident commander may approve operations above the 10% LEL action level. However, consider employing other tactics to reduce the risks to responders, including ventilation, adjusting the crew size and location, and hoseline placement and use. Adopt and train to department policy that sets action levels at hazmat incidents to maximize responder safety.
Sensor Limits, Performance, and Cross-Sensitivities
Each sensor has its own specifications, contained in the technical notes from the meter manufacturer. Our manufacturer provides the following information:
- The concentration range that the sensor can detect. This is important because the sensor could be saturated and you may be in a much higher concentration than the sensor is able to display. Some CO sensors read only up to 500 ppm; others can read up 2,000 ppm; if you enter a residence and the meter immediately pegs to 500 ppm (the sensor’s upper display limit), the CO concentration is likely much higher.
- The specific sensors installed in your meter and the upper limit display capability.
- The temperature and humidity range within which the sensor is designed to operate. If operating outside of these ranges, the sensor may not work as designed and may provide inaccurate readings.
- Response time for the PID, LEL, and O2 sensors, because for an accurate reading, a space must be metered for a certain amount of time according to the technical note. The response time is typically 15 to 30 seconds.
- A chart revealing cross-sensitivities for each sensor. This chart will tell you that if you have a reading on the meter but you know the gas you are metering is different, the number you are getting on that particular sensor corresponds to a different concentration of the cross-sensitive gas. For your CO sensor, you need to know whether the sensor inside the meter is equipped with a charcoal filter, since this changes the number for cross-sensitivity and is part of the chart. For example, a cross-sensitivity of the CO sensor found in the chart would be for hydrogen gas. If the CO sensor is reading 100 ppm but it is known that the atmosphere contains hydrogen gas, you are actually in 40 ppm of hydrogen for our department’s meter. It is not practical to memorize these values, but you must understand that the sensor could be reading something other than what it is designed for; that information is available in the manufacturer’s technical notes.
Using Multiple Technologies
In the fire service, equipment malfunctions, batteries die, and the truck’s charging unit shorts out, and now you don’t have access to one of your most crucial instruments. Even if the meter functions well at first, sometimes there are glitches—the meter doesn’t perform well, the pump is dirty, filters are clogged, a setting was accidentally changed, or a sensor needs replacement.
A multigas meter is sampling the atmosphere and running it across four to six sensors simultaneously and relies on a computer program to provide accurate results. Our department has had glitches happen on scene. If you are fortunate enough to have access to multiple meters, deploy multiple units on the scene to ensure accurate readings.
Also be aware that certain samples can damage the meter. For example, the meter is not designed to take in liquids, but users have accidentally allowed meters to intake liquid samples, damaging the sensors and the pump. Corrosive gases (ammonia, acid gases) will also damage the meter.
To provide redundancy in monitoring, use multiple monitoring units when dealing with unknown atmospheres. This is one reason hazmat response teams will also use simple chemistry such as pH paper or detector tubes vs. technology. If responders are well trained in hazmat response, these are easy-to-use items to confirm what the meters are telling us or to rule out certain compounds, as we did when our firehouse CO detectors activated. If you do not have access to alternative hazmat detection units or technologies and only have access to one multigas meter, ensure it is calibrated and serviced on schedule so you can be certain it will be ready to perform when needed.
Compounds that we cannot see or smell pose a significant risk to our health and safety. Daily human life relies on equipment that uses explosive gas; if it malfunctions, it can produce deadly gas. Detection equipment saves lives. Metering expertise will keep firefighters safe; confirm that we have mitigated an incident; and, most importantly, allow us to inform the public that they are safe.
Understanding the intricacies of our meters ensures that we are deploying them properly and interpreting their data accurately to help drive an operation no matter how small or large the incident may be. Even if a department is not equipped with a hazmat team and must rely on regional hazmat response, initially first-due companies will handle the public’s first call when people are ill, detector activations, and reports of abnormal smells. Mastering the use of the multigas meter will undoubtedly keep all responders in charge of mitigating these incidents safe from harm and enhance the quality of service we provide to our citizens.
Richard C. Beaulieu is a lieutenant on Rescue 2 for the Cranston (RI) Fire Department (CFD), where he has served since 2008. A 15-year veteran of the fire service, he has a bachelor’s degree in fire science from Providence College. A hazmat technician since 2006, Beaulieu is an NFPA 1041-certified instructor and coordinates the hazmat technician training for the CFD’s recruit training academy.